Introduction
Obesity is a multifactorial disorder that is a severe problem in westernized lifestyles owing to high-calorie diets and insufficient physical activity (Singh et al., 2022). It has been linked to a number of endocrine abnormalities caused by changes in the hypothalamic-pituitary hormone axis (Ylli et al., 2022). Also, obesity is closely associated with low-grade chronic inflammation, excessive oxidative stress, and abnormal mitochondrial function (Ruiz-Ojeda et al., 2018; Thakuri et al., 2023b). Adipogenesis and lipogenesis, which are the processes of fat differentiation and lipid synthesis in the body, play critical roles in the development of obesity (Jakab et al., 2021). The master regulators of adipogenesis, peroxisome proliferator activator receptor-γ (PPAR-γ) and CCAT/enhancer-binding protein-α (C/EBP-α), build adipocytes that lead to lipogenesis by regulating lipogenic factors such as sterol regulatory element-binding transcription factor 1c (SREBP-1c) and fatty acid synthase (FAS). In addition, AMP-activated protein kinase (AMPK), sirtuin-1 (SIRT1), leptin, and adiponectin are known to play essential roles in regulating the balance of fat metabolism (Fontecha-Barriuso et al., 2020; Salminen et al., 2011). Therefore, it is very important to understand the relationship between these indicators to improve metabolic diseases such as obesity and type 2 diabetes. For this reason, drug development based on these mechanisms has recently received attention. However, due to the side effects of drugs, the use of functional foods and natural products is emerging as an alternative treatment. Functional foods contain biologically active ingredients that are associated with physiological health benefits for the prevention of metabolic diseases (Alkhatib et al., 2017; Green et al., 2020).
Abalone, a gastropod mollusk found in coastal saltwater, is considered a culinary delicacy (Bullon et al., 2022). It is one of the most commercially available fishery resources in Asia (Cook, 2023). Pacific abalone Haliotis discus hannai is commercially valuable in South Korea (Park & Kim, 2013). Previous studies have reported its antitumor, antioxidant, and anti-inflammatory properties (Nguyen et al., 2010, 2014; Shin et al., 2022; Zheng et al., 2019). Some studies have also demonstrated that abalone is a good source of iron, vitamins, fat (omega-3), proteins, and amino acids, such as arginine and taurine (Bullon et al., 2022; Lee et al., 2023). However, industrial use and research on abalone intestine are lacking. Nevertheless, abalone intestine is rich in nutrients and bioactive ingredients, which might be due to the ingestion of food sources such as the seaweed known as kelp, Saccharina japonica (SJ) (Troell et al., 2006; Yu et al., 2022). Studies have reported the kelp contains physiologically active compounds, such as alginic acid, polysaccharide sulfate (fucoidan), and glutamic acid (Bullon et al., 2022). A previous study also reported that the abalone adult intestine contained protein (19.19%), moisture (70.14%), lipid (1.2%), carbohydrate (4.01%), ash (5.46%) after food digestion (Qian et al., 2012). However, nutritional values may also vary due to endogenous factors such as animal genetics, age, and sex (Wang et al., 2023). Therefore, the ultimate purpose of this study is to investigate the effect of abalone intestine extract on fat metabolism and body weight control, and to determine whether the differences of abalone’s food source, male or female, and extraction method are correlated with efficacy.
Materials and Methods
The Cell Counting Kit-8 (Dojindo Laboratory, Kumamoto, Japan), 3-isobutyl-1-methylxanthine (IBMX), 2,2-diphenyl-1-picrylhydrazyl (DPPH), dexamethasone, insulin, Folin-Ciocalteu reagent, gallic acid (GA), chicken egg yolk, and Oil Red O, were purchased from Sigma-Aldrich (St Louis, MO, USA). Antibodies against PPAR-γ (sc-7273), C/EBP-γ (sc-365318), SREBP-1c (sc-365513), FAS (sc-48357), and β-actin (sc-47778) were purchased from Santa Cruz Biotechnology (Dallas, TX, USA), and phospho-AMPK (Thr172) (#2535), AMPK (#2532), anti-mouse IgG (#7076) and anti-rabbit IgG (#7074) were purchased from Cell Signaling Technology (Danvers, MA, USA). The protein assay dye, acrylamide/bis solution, polyvinylidene (PVDF) membrane, and all reagents used for western blotting were purchased from Bio-Rad (Richmond, CA, USA). Chemiluminescent substrates were purchased from Thermo Fisher Scientific (Waltham, MA, USA). Enzyme-linked immunosorbent assay (ELISA) kits were purchased from Asan Pharmaceutical (Seoul, Korea). Sodium carbonate, methanol, and ethanol were purchased from DUKSAN (Ansan, Korea).
The intestines of male and female abalone (MA and FA) used in this experiment were provided by Professor Lim Han Kyu of the Department of Marine and Fisheries Resources at the Mokpo National University.
The sample was extensively washed with tap water, dried in a hot air dryer for 24 h, and then powdered to facilitate extraction. For the hot water extract (water), sample powder (4 g) was immersed in 200 mL of distilled water, stirred, extracted at 95°C for 4 h, and filtered through a Buchner funnel using 110 mm Whatman filter paper. The solution was freeze-dried (freeze dryer, Operon, Gimpo, Korea) and stored at –98°C. For the ethanol extract (EtOH), sample powder (4 g) was immersed in 200 mL EtOH, followed by stationary extraction at room temperature for 24 h. After 24 h, filtration was performed using a No. 2 Whatman filter paper. After concentration under reduced pressure at 37°C, the solution was freeze-dried and stored at –98°C. For the preparation of the subcritical water extract (SW), 4 g of the sample (MA, FA, and SJ) and 200 mL of tertiary distilled water were mixed and kept in the reaction cell of a subcritical water reactor (TPR-I, T-04231, Taiatus Techno, Tokyo, Japan). The temperature was adjusted to 180°C or 210°C with a constant pressure of 3 MPa for 10 s. After the reaction, the extracts [SW at 180°C (SW180) or SW at 210°C (SW210)] were allowed to cool to room temperature, and the reaction solution in the reaction cell was collected and filtered under reduced pressure
(No. 2, 110 mm filter paper, Whatman, Buckinghamshire, UK), followed by freeze-drying. The final powdered extracts were stored at –20°C. The collected yields were as follows: MA extracts [water 1.17 g (29.4%), EtOH 0.48 g (11.9%), SW180 1.88 g (47.0%), and SW210 2.46 g (61.5%)], FA extracts [water 1.41 g (35.30%), EtOH 0.50 g (12.4%), SW180 2.12 g (53.0%), and SW210 2.13 g (53.1%)], and 1.20 g of SJSW210 (30.0% yield).
The total polyphenol content was measured using the Folin-Ciocalteu method with some modifications (Rumbaoa et al., 2009). Briefly, 0.1 mL of Folin-Denis reagent was added to the sample solution diluted 2,000 times with distilled water, mixed, and reacted at room temperature for 30 s. After, 0.3 mL of 20% sodium carbonate solution were mixed and allowed to react for 2 h at room temperature. The absorbance was then measured at 765 nm using a spectrophotometer (Immuno Mini NJ-2300, Biotech, Tokyo, Japan). The total polyphenol content was obtained using a standard curve of gallic acid (Yakuri Pure Chemicals, Osaka, Japan) at concentrations of 0.03125, 0.0625, 0.125, 0.25, and 0.5 mg/mL dissolved in distilled water.
3T3-L1 preadipocytes (ATCC, Manassas, VA, USA) were cultured in Dulbecco’s modified Eagle’s medium (DMEM) containing 10% newborn calf serum and 1% penicillin-streptomycin. For cell differentiation, 3T3-L1 preadipocytes were grown until confluence, and the medium was replaced with a differentiated medium containing 10% fetal bovine serum (FBS) and MDI (0.5 mM IBMX, 1 μM dexamethasone, 5 μg/mL insulin) for 2 days. Next, the medium was replaced with fresh medium containing 5 μg/mL insulin and DMEM medium containing 10% FBS and changed on every 2 days for 8 days in a humidified incubator, at 37°C with 5% CO2 until cells were differentiated.
3T3-L1 preadipocytes at a density of 1 × 104 cells/well were cultured into a 96-well plate. Different abalone intestine extracts at the concentrations of 250 µg/mL were treated during differentiation (0–8 days). On each well, 100 µL of water-soluble tetrazolium salt (WST) solution (1 mg/mL) were added and incubated for 2 h in CO2 incubator. After that, an optical density was measured at a wavelength of 450 nm (Immuno Mini NJ-2300, Biotech).
To evaluate the inhibition of lipid accumulation, samples were administered during the differentiation of 3T3-L1 cells. On day 8, fully differentiated 3T3-L1 adipocytes were fixed with 10% formalin for 30 min after washing twice with 1 mL of 1X phosphate-buffered saline (PBS) (Gibco, Thermo Fisher Scientific) at room temperature. Next, the cells were stained with Oil Red O solution (0.5% in 60% isopropanol) for 1 h. Finally, the cells were washed with 60% isopropanol and distilled water. Lipid droplets in 3T3-L1 adipocytes were examined by phase-contrast microscopy. To measure the triglyceride content (TGC), stained cells were incubated with 100% isopropanol for 15 min at room temperature, and the colored solvents were transferred to a 96-well plates, where optical densities were measured at a wavelength of 540 nm.
To determine protein expression, cell lysates containing 15 µg of protein were separated in a 10% SDS-PAGE and transferred to a PVDF membrane. The PVDF membrane was blocked with 5% skim milk or 5% bovine serum albumin for 1 h, rinsed in TBS-T buffer (1 mmol/L Tris, 5 mmol/L NaCl, and 0.1% Tween-20; pH-7.6), and incubated overnight with anti-PPAR-γ (1:250), C/EBP-α (1:250), SREBP-1c (1:250), FAS (1:250), p-AMPK (1:1,000), AMPK (1:1,000), and β-actin (1:2,000) primary antibodies. The PVDF membrane was again washed with TBS-T buffer, followed by incubation with a secondary antibody (horseradish peroxidase-conjugated anti-rabbit or anti-mouse and rabbit IgG [1:1,000]) for 1 h at room temperature. Finally, the expressed proteins were examined using a chemiluminescent substrate.
In a flow-through system (Zebtec, Techniplast, Buguggiate, Italy), wild-type adult zebrafish (Danio rerio, AB line) were cultivated under a 14 h light/10 h dark cycle at a temperature of 28°C with a pH of 7.0. According to the zebrafish developmental stage, different sizes of Gemma Micro zebrafish feed (Skretting Zebrafish, Tooele, UT, USA) were administered twice or thrice daily. Adult male and female zebrafish were paired in mating tanks at a 1:1 ratio and the embryos were harvested the following day. The healthy embryos were cultured in a 96-well plate with zebrafish embryonic medium (E2) until 6 days’ post fertilization (dpf). At 6 dpf, the zebrafish larvae were treated with samples at a concentration of 250 µg/mL and incubated for 24 h. Larvae were checked for toxic effects by observing malformation and mortality under the microscope, and the rate was calculated as previously described (Thakuri et al., 2023a). All studies were carried out in accordance with the laboratory animal care standards established by the Mokpo National University.
For obese zebrafish, a previously described protocol (Den Broeder et al., 2017) was followed with some modifications. Briefly, at 5 dpf, zebrafish larvae were preselected, transferred to a petri dish and divided into four groups, including a control group. Each petri dish (150 × 25 mm) contained 50 mL of E2 with 40 larvae in each group. Gemma Micro feed was fed to control group, and 1 mg/mL chicken egg yolk (Sigma-Aldrich) diluted with E2 medium (final concentration 0.4%) was administered to the HFD group. Samples at a concentration of 250 µg/mL were added to the HFD solution until 10 dpf. All feeds were prepared and changed according to the required final volume and concentration.
Because zebrafish larvae weigh very light, a pool of 10 larvae was used to measure body weight. Body weight was calculated by removing the water from the pool (Thakuri et al., 2023c). To measure glucose levels, 30 larvae from each group were homogenized using Bullet BlenderTM (Next Advance Lab Products, Palmer, Troy, NY, USA) following the manufacturer’s protocol in a capped-microcentrifuge tube with zirconium oxide beads (0.5 mm). After homogenization, the supernatants were collected in fresh tubes, and glucose levels were measured using a guideline provided with glucose assay kit (AM201, Asanpharm, Seoul, Korea).
Zebrafish larvae were fixed overnight with 4% paraformaldehyde at 4°C and washed three times with PBS. Subsequently, the zebrafish larvae were immersed in 85% and 100% 1,2-propanediol at room temperature for 20 min. Then, fresh 0.5% Oil Red O solution was added, and zebrafish larvae were dyed at room temperature for 4 h. The zebrafish larvae were washed with 100 and 85% 1,2-propanediol, respectively. Finally, lipid droplets in the liver tissue were observed and photographed using a fluorescence microscope. Images of the larvae were captured using a LEICA MZ10F microscope (Leica, Wetzlar, Germany) equipped with a mounted camera and iSolution software.
Total RNA was extracted from a pool of 40 larvae homogenized using the Bullet BlenderTM homogenizer and isolated using TRIzol reagent (Molecular Research Center, Cincinnati, OH, USA). The RNA primers were purchased from Integrated DNA Technologies (Coralville, IA, USA). Quantitative RT-PCR (qRT-PCR) assays were conducted on the Applied Biosystems StepOneTM Real-Time PCR System Thermal Cycling Block (Thermo Fisher Scientific) using the SYBR Green Real-Time qPCR Kit (BIOFACT, Daejeon, Korea). The sequence of the oligonucleotide primers shown in Table 1. The qPCR reaction conditions were: 15 min at 95°C, followed by 40 cycles of 15 s at 95°C, 45 s at 55°C–60°C, and plate analysis. The mRNA expression levels were calculated using the 2−∆∆Ct (Ct, threshold cycles) method and normalized against an endogenous control, glyceraldehyde-3-phosphate dehydrogenase (GAPDH).
Data are shown as the mean and standard error of the mean (SE). All statistical analyses were performed using GraphPad Prism version 8.3, and one-way analysis of variance followed by Dunnett’s test or Tukey multiple comparison test (GraphPad Software, San Diego, CA, USA). Statistical significance was set at p < 0.05.
Results
The contents of MA and FA extracts changed in the following order: SW210 > SW180 > water > EtOH (Fig. 1A). Among SJSW210, MA210 and FASW210, FASW210 showed the highest phenol content [11.30 mg equivalent of gallic acid (GA)/100 g of extract] (Fig. 1B).
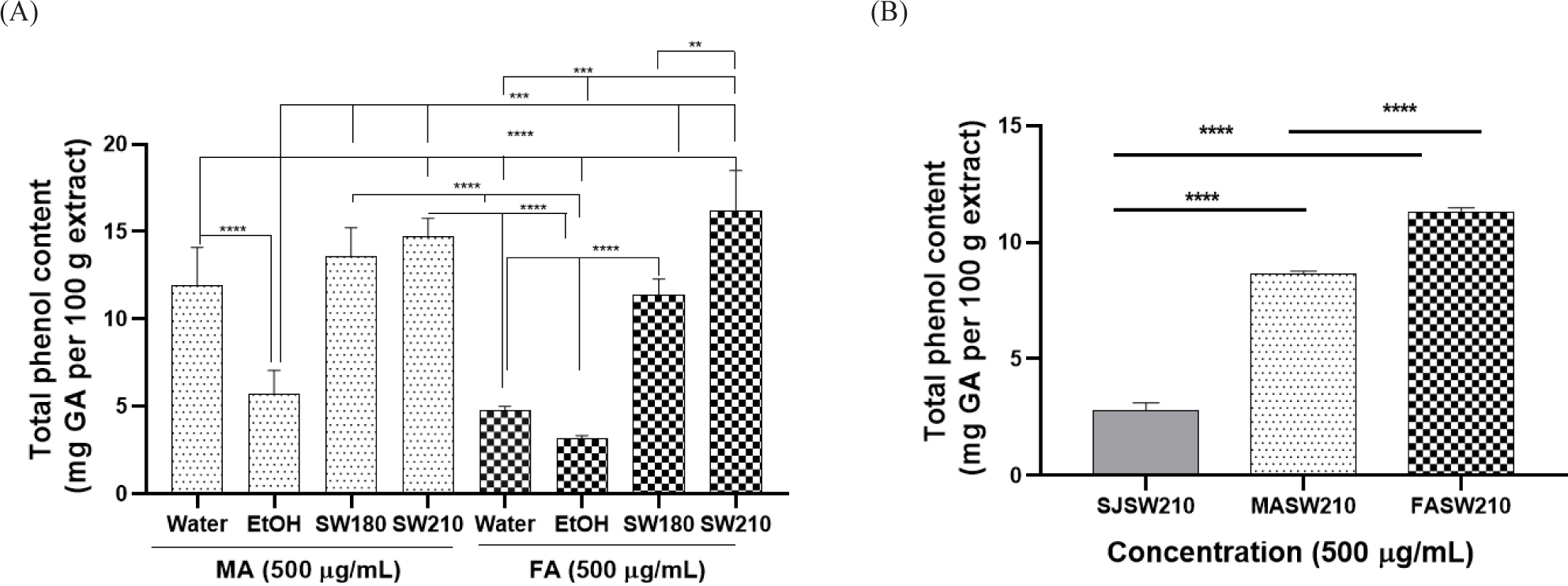
None of the extracts showed cytotoxicity at concentrations up to 250 µg/mL in 3T3-L1 adipocytes (Fig. 2). Lipid accumulation examined by Oil Red O staining, as shown in Figs. 3 and 4, revealed that MASW210 at a concentration of 250 µg/mL showed the greatest inhibition of lipid accumulation (39%) compared to the control or other groups (SJSW210 and FASW210).
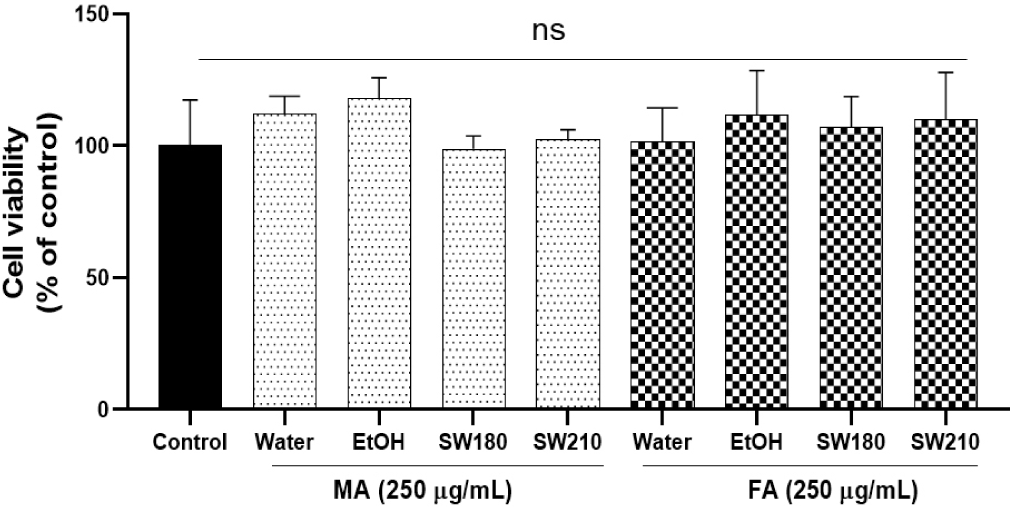
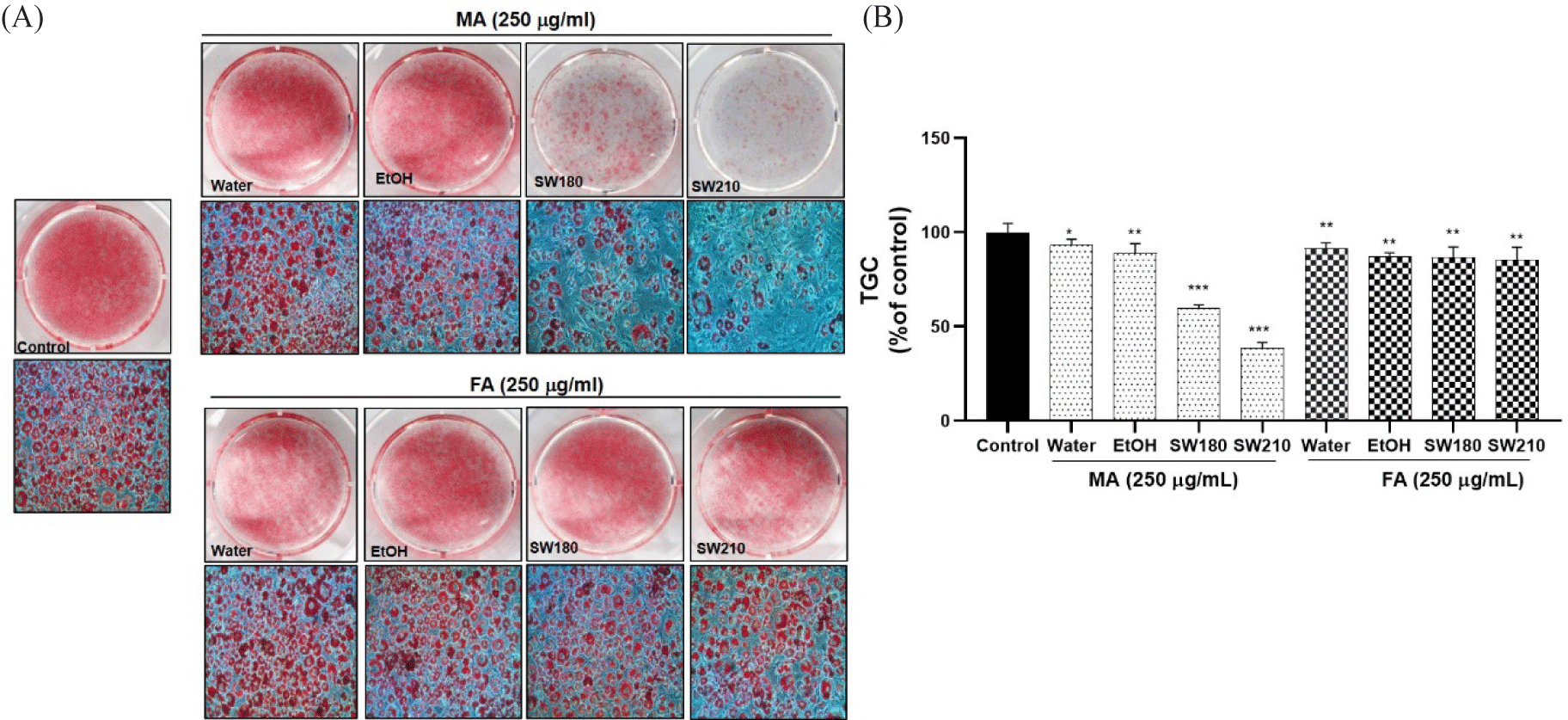
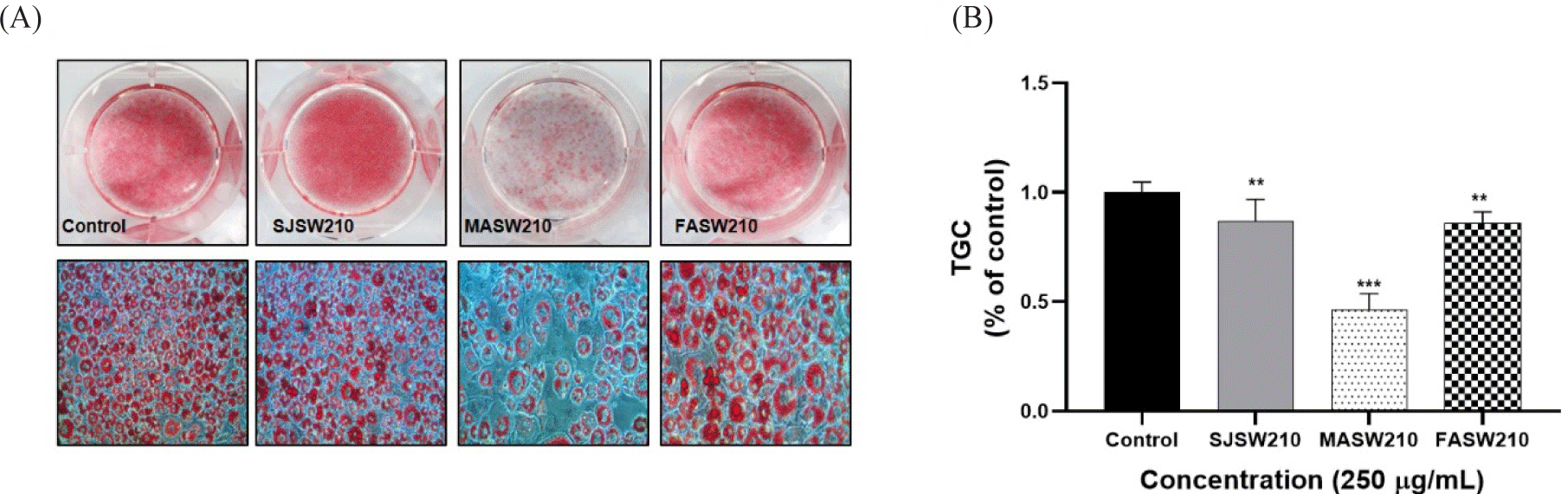
Expression of the key activators of adipocyte differentiation such as PPAR-γ and C/EBP-α was significantly decreased in MASW210 treated group compared to control or other extracts (Fig. 5A and 5B). In addition, MASW210 significantly increased AMPK activity, which is a major factor in cellular energy homeostasis (Fig. 5C). AMPK is a well-known regulator of adipogenesis in adipocytes (Göransson et al., 2023). As shown in Fig. 6, the protein expression of both SREBP-1c and FAS, which are closely related to lipogenesis, was significantly reduced by the addition of MASW210 compared with that in the other groups. Since MASW210 strongly inhibited lipid accumulation, further experiments were performed using only MASW210.
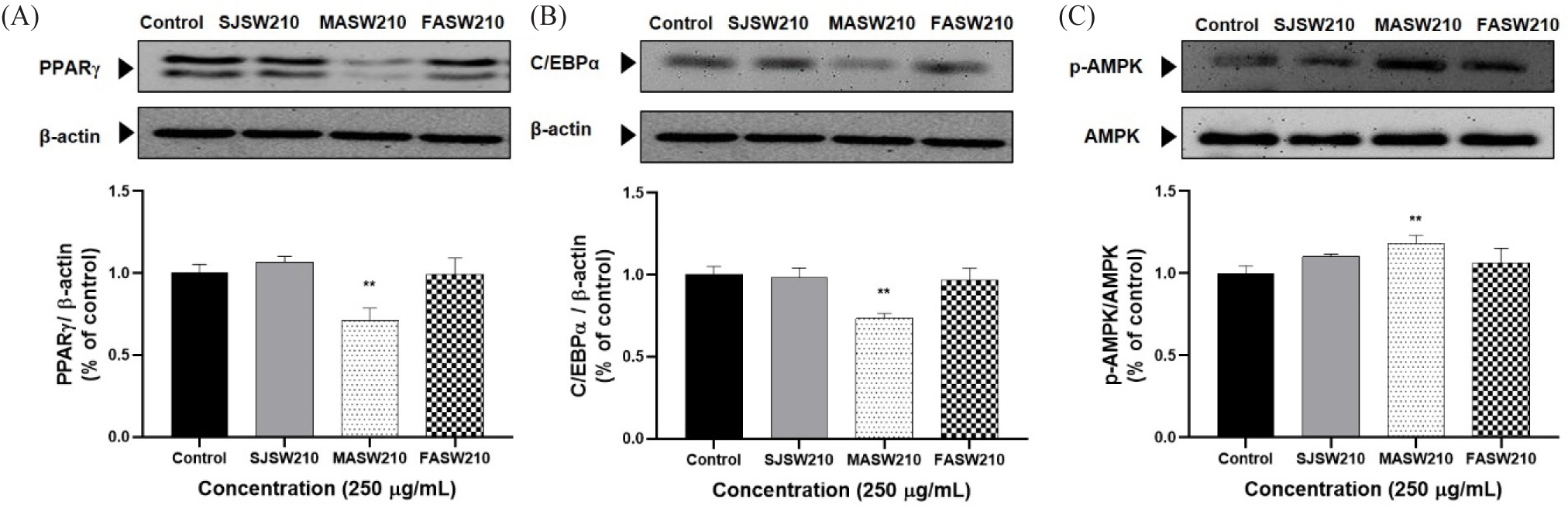
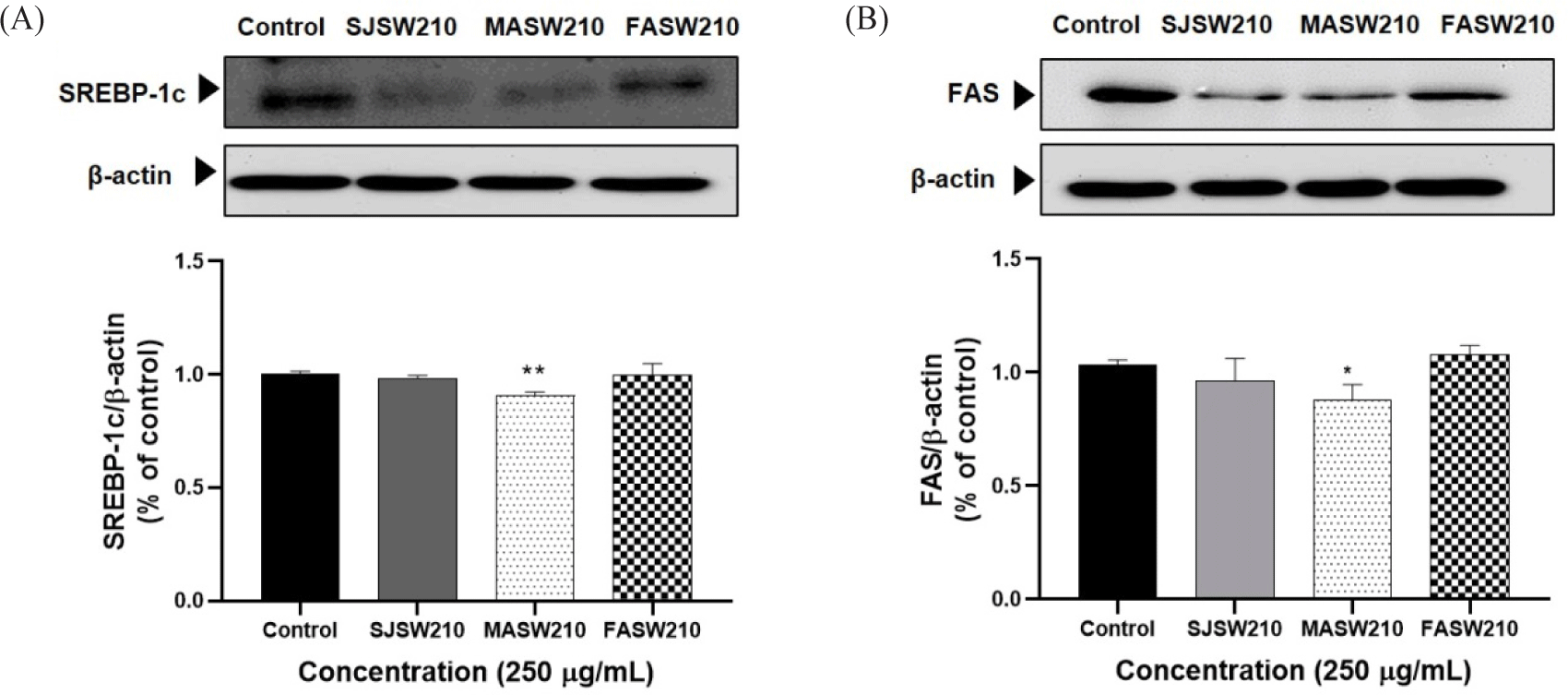
As expected, the HFD-treated group showed a significant increase in weight (1.5 fold approx. compared to the control), and glucose levels (152 mg/dL), a hallmark of insulin resistance compared to the control group (Fig. 7A and 7B). Treatment with SJSW 210 or MASW210 drastically reduced weight gain (Fig. 7B). Similarly, elevated glucose levels in the HFD-fed group were significantly decreased by the addition of SJSW210 or MASW210, which was clearly observed in the MASW210 group (Fig. 7C). During staining, Oil Red O is transferred from the dye solution to fat, so that the lipid droplets can be stained red (Du et al., 2023). Interestingly, the lipid around the abdomen of larval zebrafish increased more obviously (Fig. 7D), where we speculate it as the location of liver tissue according to the anatomy of zebrafish. The sensitivity of Oil Red O staining around the liver sections of HFD larvae confirmed a gradual decrease in lipid accumulation in the SJSW210 and MASW210 groups. These findings demonstrate the anti-obesity effects of male abalone intestinal subcritical water extract.
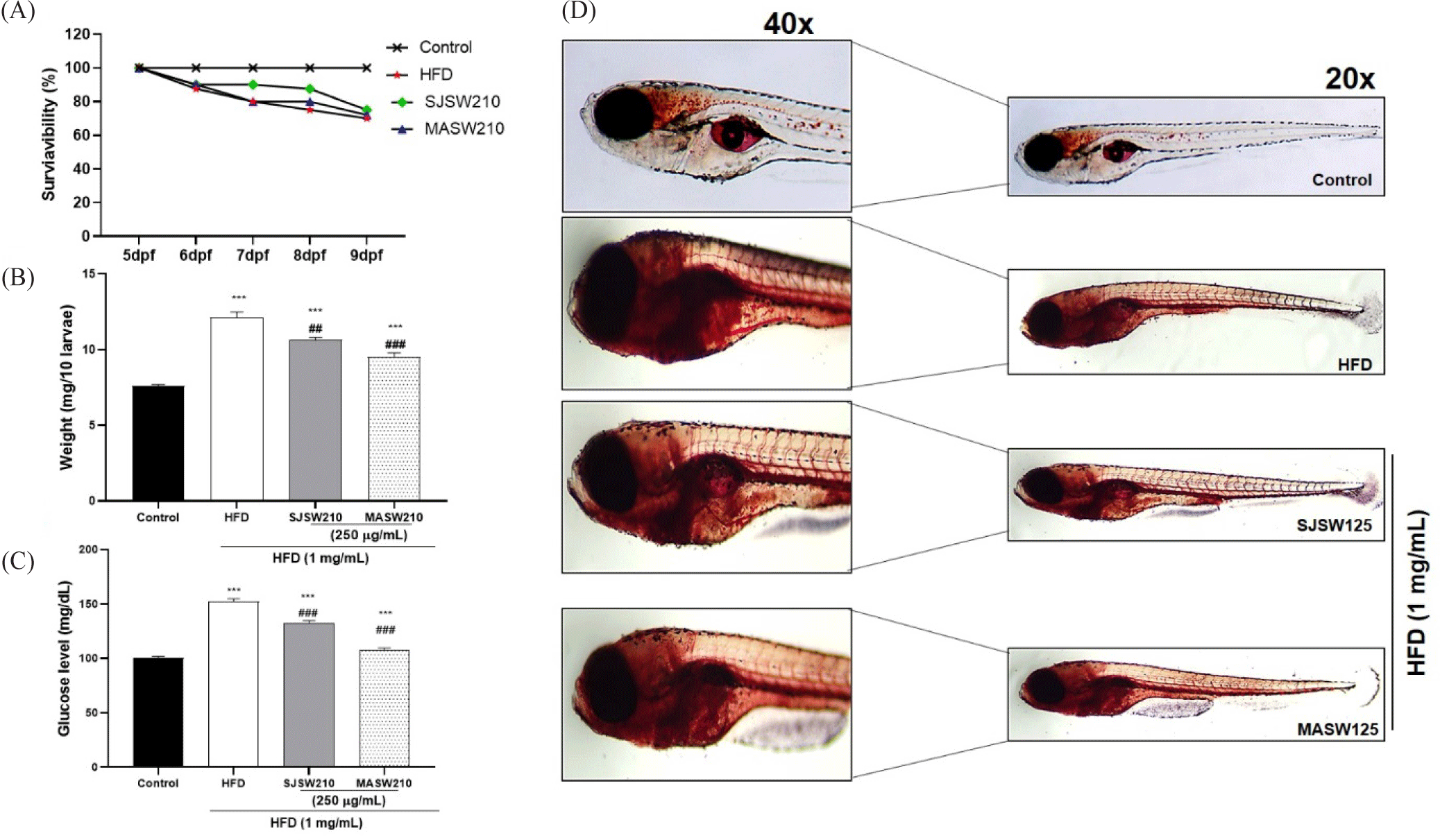
Furthermore, mRNA expression was investigated to study the mechanism of action of MASW210 in obesity-associated metabolic syndrome. Compared with the control group, HFD-induced obese larvae showed an increase in adipogenic or lipogenic transcriptional factors PPAR-γ (54%), C/EBP-α (49%), FAS (30.2%), and FABP (75%) (Fig. 8A–8D). However, the expression of these genes was significantly reduced by MASW210 treatment, and was more effective than SJSW210. In addition, the mRNA expression of pro-inflammatory cytokines, such as TNF-α and IL-6, was increased by HFD, which was also abbreviated by MASW210 treatment (Fig. 8E and 8F). PGC-1α and CPT-1 play important roles in fatty acid oxidation and mitochondrial biogenesis (Wang et al., 2020). MASW210 markedly increased the mRNA expression levels of these genes (Fig. 9). These data suggested that MASW210 promotes mitochondrial biogenesis. Similarly, the HFD group showed downregulation of AMPK, lepr, sirt1, and adiponectin expression compared with the control group, but it was significantly increased in both sample groups (SJSW210 or MASW210). The SJSW210 group showed a level similar to that of the control group; however, MASW210 showed a greater increase than that of the control group (Fig. 10A–10D).
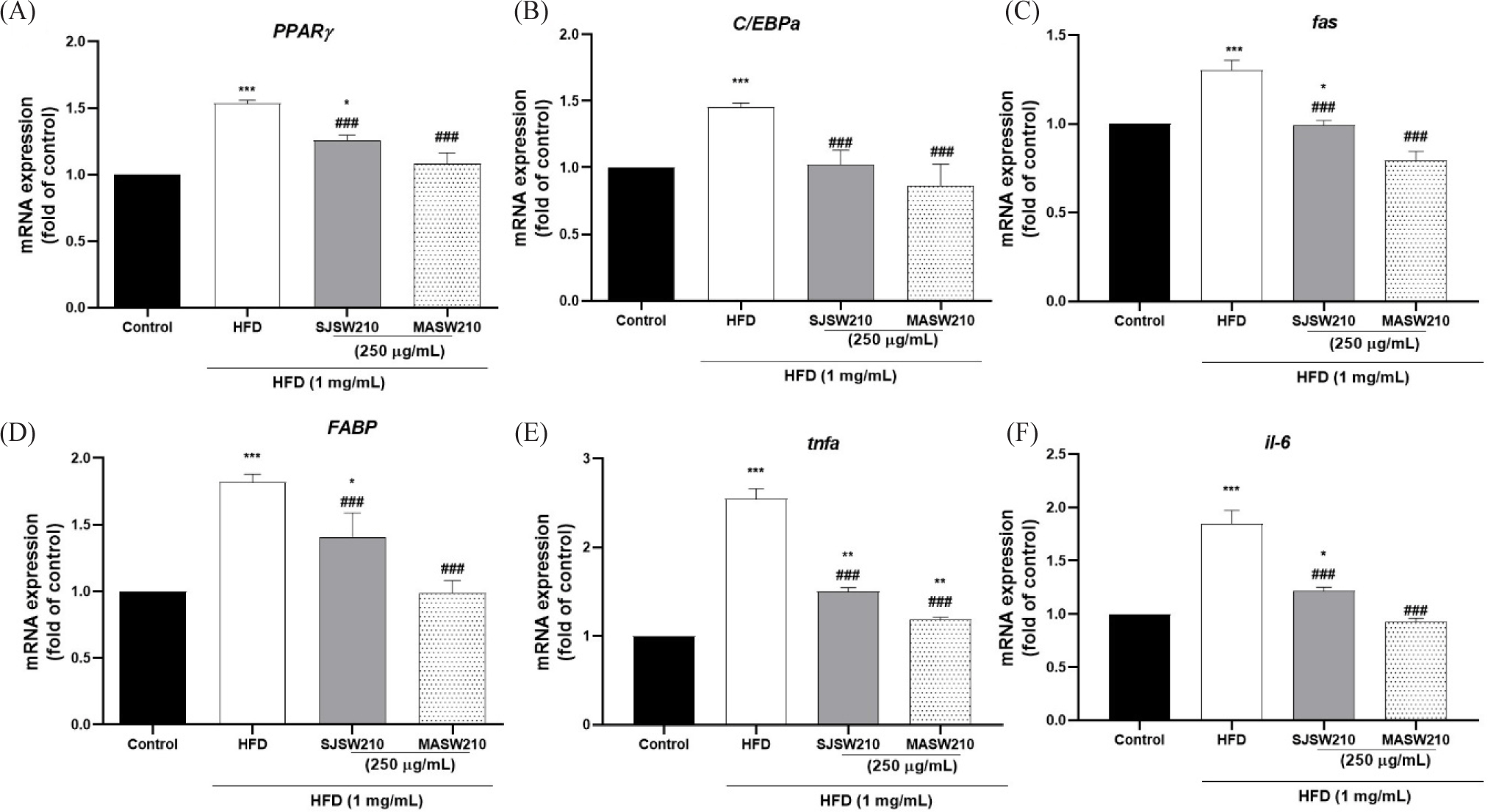
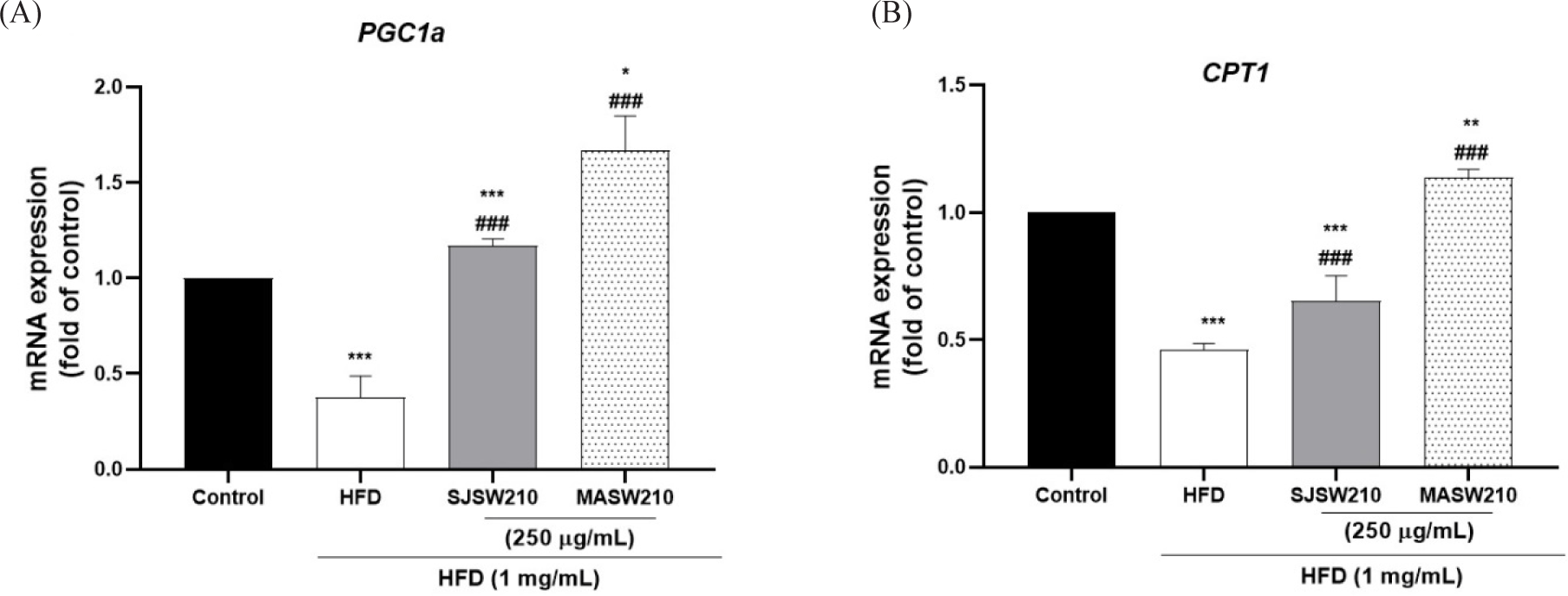
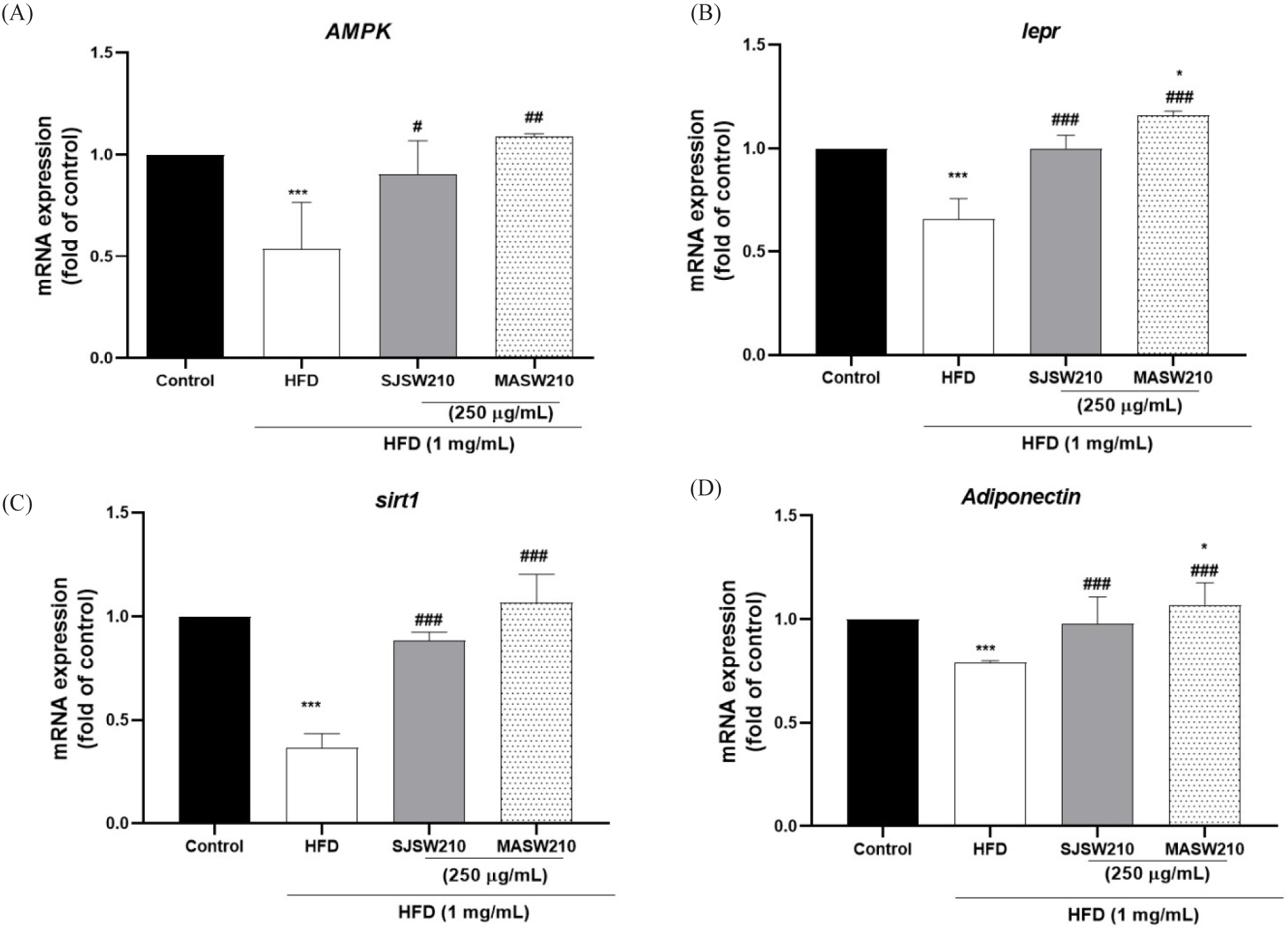
Discussion
Abalone is a safe marine organism consumed as a food source and has many nutraceutical and therapeutic benefits (De Zoysa, 2013; Khan & Liu, 2019). Abalones break down valuable components into simple molecular structures that can be exploited as nutraceutical resources (Bullon et al., 2022; Cheng et al., 2023). Therefore, the effects of MA and FA intestines, with kelp as a dietary source, on fat metabolism and metabolic symptoms were compared. MASW210 significantly suppressed fat synthesis and related gene expression in 3T3-L1 cells and zebrafish larvae. It also effectively controlled the mRNA expression related to inflammation, energy metabolism, and mitochondrial regulators in HFD-induced zebrafish larvae. The results demonstrated that MASW210 modulates the pathophysiology of obesity and metabolic disorders.
SWE is a green technique for extracting phytochemicals with high biological activities from extracts and phytochemicals such as phenolic compounds (Romulo, 2020; Thakuri et al., 2023b, 2023c). Phenolic compounds are vital for defense responses such as antioxidant, anti-inflammatory, and anti-aging activities (Lin et al., 2016; Rahman et al., 2021). Scientific reviews have reported that, under optimal conditions, SWE extracts unstable polyphenols and polyphenolic compounds that are positively correlated with antioxidant activity (Cheng et al., 2021). These compounds have been reported to reduce physiological weight gain and prevent obesity by decreasing adipogenesis and regulating lipid metabolism (Shin et al., 2022; Yen et al., 2020). Consistent with previous findings, the SWE of abalone intestine contained the highest total phenol content (Fig. 1), and MASW210 showed the strongest inhibitory effect on lipid accumulation in 3T3-L1 adipocytes compared to other extracts (Fig. 3). Additionally, the regulatory effect of MASW210 on abnormal fat metabolism was related to the significant suppression of adipogenic and lipogenic transcription factors, such as PPAR-γ and C/EBP-α, SREBP-1c, and FAS, in both 3T3-L1 adipocytes and HFD-induced zebrafish larvae (Figs. 5, 6, and 8). Therefore, our results revealed that SWE is an effective technology for extracting polyphenol components and that MASW210 is more favorable for fat metabolism than FASW210 and SJSW210.
Obesity is characterized by low-grade chronic inflammation that leads to the increased production of pro-inflammatory mediators, including TNF-α and IL-6 (Thakuri et al., 2023b). Excessive adipokines production by adipose tissue is known to induces mitochondrial dysfunction and oxidative stress (Li et al., 2023). These factors promote the downregulation of PGC1α and CPT1 expression, which is correlated with metabolic dysfunction, mitochondrial biogenesis, and fat metabolism (Kang et al., 2018; Kwon et al., 2020). As expected, the mRNA expression of TNF-α and IL-6 was increased more than two fold by HFD in zebrafish larvae, and the mRNA expression of PGC1α and CPT1 associated with mitochondrial dysfunction was reduced by more than twofold compared to that in normal larvae (Figs. 8 and 9). However, MASW210 effectively restored the expression of genes related to inflammatory adipokines and mitochondrial metabolic disorders to levels similar to those observed in the normal larvae. Thus, our results emphasize that MASW210 restores the abnormal energy metabolism and adipokines production caused by excessive nutrient intake.
Finally, we measured the function of SIRT1, lepr, AMPK, and adiponectin in HFD-induced obese zebrafish larvae, as these factors have been reported to play a role in weight control and metabolic regulation (Grahame Hardie et al., 2012; Lee et al., 2020). Several studies have reported that SIRT1 activation decreases inflammatory cytokine levels and leptin and adiponectin regulate metabolic homeostasis (Mariani et al., 2020). Leptin, a hormone secreted by fat cells, acts as a mediator of inflammation and energy metabolism by activating lepr, which regulate lipid metabolism, blood glucose, and insulin secretion (Obradovic et al., 2021; Zhang et al., 2018). Many studies have shown that AMPK activation reduces plasma glucose and lipid levels, inhibits excessive fat synthesis, and increases insulin sensitivity, thereby reducing obesity and related diseases (Long & Zierath, 2006; Wang et al., 2021). Adiponectin is a hormone secreted by adipose tissue that has various metabolic effects that improve insulin sensitivity, reduce inflammation, and promote fatty acid oxidation (Achari & Jain, 2017; Kojta et al., 2020). Therefore, understanding the functions of SIRT1, lepr, AMPK, and adiponectin in controlling weight gain and obesity could shed light on prospective therapeutic approaches for the treatment of obesity and related metabolic diseases (Roy & Palaniyandi, 2021; Wen et al., 2022). Here, the expression of these genes was significantly decreased in HFD-induced obese zebrafish larvae compared to that in normal larvae, but MASW210 restored their mRNA expression to normal levels. These results suggested that MASW210 positively affects body weight and fat metabolism by regulating energy and metabolic homeostasis. Although the effect of MASW210 on the fat metabolism and metabolic homeostasis has been acknowledged in this study, lots of issues need to be clarified in the future, especially, further research is required to identify potential active ingredients that have the strongest physiological effects on metabolic syndrome.