Introduction
New challenges arise in the face of global climate change that affects every ecosystem on earth, including the aquatic environment, which causes accelerated loss of biodiversity in several aquatic ecosystems (Worm & Lotze, 2021). Climate change is expected to cause major changes in water scarcity in some areas and an increased risk of flooding in most areas. In addition, climate change has increased the temperature of the waters, thus affecting water quality and freshwater ecosystems (Mann et al., 2022). Several studies have shown clear evidence that biodiversity is already responding to climate change (Gillette et al., 2022). Apart from climate change, other anthropogenic impacts also affect freshwater ecosystems (Häder et al., 2020).
Fish comprise 50% of the total number of existing vertebrate species and are widely recognized for their notable variation in species diversity. Hence, fish comprise a significant portion of the taxonomic diversity within the animal kingdom. Moreover, these organisms hold significant significance as primary sources of animal protein for human consumption, thereby possessing considerable economic worth. Nevertheless, the presence of human disturbances renders the richness and abundance of fish biodiversity in aquatic ecosystems increasingly susceptible. The study reported that the extinction rate of fish in fresh waters was higher (74.2%) compared to 47.4% for marine fish (Wiens, 2016). The negative impacts caused by human activities, including deforestation, river damming, overfishing, water contamination from plastic and domestic waste, illegal hunting, and degradation of habitats, have led to a significant decline in the diversity of fish species (Giannetto & Innal, 2021).
The identification and classification of fish species commonly rely on morphometric and meristic characteristics. Nonetheless, it is important to note that morphological characteristics may not exhibit consistent stability across different developmental stages. Additionally, the assessment of incomplete samples or the evaluation of rare and cryptic species often presents challenges. Moreover, the process of fish identification can present challenges owing to the resemblance in physical characteristics among closely related species during their early developmental stages, as well as inconsistencies found in the available literature and taxonomic records. These difficulties persist even when examining complete, fully-grown individuals. Furthermore, it is important to acknowledge that taxonomists may possess diverse levels of proficiency and expertise in identification. As a result, there is a possibility of inconsistent identification of the same specimen by different taxonomists, leading to potential confusion when attempting to summarize and compare data (Hulley et al., 2019). Nevertheless, environmental and conservation studies require a high degree of precision, necessitating the identification of specimens at the species level (Krishna Krishnamurthy & Francis, 2012). Because of the fundamental constraints of alpha taxonomy and the decreasing number of taxonomic experts, genetic techniques for fish species identification are required (Hopkins & Freckleton, 2002).
Molecular identification, which uses molecular markers to identify species, is commonly employed today. DNA barcoding, which relies on the analysis of mitochondrial DNA (mtDNA), is considered a highly appropriate method for species-level identification when compared to other molecular approaches commonly employed for species-level molecular identification (Panprommin et al., 2020). As opposed to morphological techniques, mtDNA-based molecular identification has various benefits. In the context of species identification, it is not imperative to possess complete specimens. Instead, a minute portion of tissue is appropriate for DNA extraction (Tapilatu et al., 2021). Furthermore, DNA is more durable and resistant to deterioration than physical characteristics (Moran et al., 2016), and some species with identical physical traits, such as cryptic or sister species, are difficult to recognize (Melo et al., 2016). Molecular identification can aid in the proper differentiation of such species (Feng et al., 2019). In addition, DNA remains constant throughout an organism’s developmental phases. Morphological characteristics, on the other hand, might alter throughout a life cycle, leading to species misidentification (Tillett et al., 2012). Consequently, molecular techniques are able to be used to identify from the embryonic stage of fish to adult forms (Rathnasuriya et al., 2021). Being a professional conventional taxonomist takes a significant amount of patience, effort, and materials (Godfray, 2002). In addition, technological advances have made it relatively easy to analyze DNA sequences, coupled with the ability of bioinformatic applications can autonomously compare them, the level of training necessary for molecular identification is considerably lower compared to that required for morphological classification. Molecular identification is used extensively in many fields apart from species identification, such as the fight against wildlife trafficking and food fraud, the detection of biological invasions, and the tracking of biodiversity (van Der Heyde et al., 2020). The cytochrome oxidase subunit I (COI) can function as a DNA barcode for biological verification across invertebrates and vertebrates (Francisco et al., 2022). Researchers from various scientific institutions worldwide have collaboratively established the Fish Barcode of Life Initiative (FISBOL) in order to create a comprehensive repository of DNA barcodes for every fish species, thereby establishing a standardized reference library (Becker et al., 2011).
The complexity of problems in Indonesia’s freshwater biodiversity, including global warming, increasing human population, riverbank damming, overconsumption/exploitation, pollutants, and invasive alien species, has led to a decline in freshwater biodiversity. Furthermore, it is estimated that 1,275 fish species in Indonesia have been listed as endangered (IUCN, 2022).
The Batanghari River is recognized as one of the most extensive rivers situated on the island of Sumatra. It flows through the provinces of Jambi and West Sumatra and has seven major river branches that contain tributaries and lakes that serve as fish juvenile breeding grounds. Geographically, it is located between 1°10'50.6"S 101°59'24.3"E and 1°23'12.4"S 103°59'08.1"E (http://www.geomapapp.org). The current state of the Batanghari River is alarming, as it is not only polluted by mercury-containing illegal gold mining waste (PETI) and industrial waste but also by household waste (Kaban et al., 2020). Consequently, several fish species in the Batanghari River have been declared extinct, with populations declining to rare or endangered levels (IUCN, 2022). A prior investigation revealed that assessments of fish populations were carried out in the Batanghari River, Sumatra, spanning the years 1994 to 2003. A total of 297 fish species in the Batanghari River, with 48 of them being newly documented records (45 of which were previously unrecorded in Sumatra). A total of six novel species have been documented under the taxonomic groups Cyprinidae, Nemacheilidae, and Cobitidae. The species Diplocheilichthys, Diplocheilichthys jentinkii, Crossocheilus pseudobagroides, Osteochilus scapularis, Osteochilus vittatoides, Rasbora hosii, and Leptobarbus rubripinna have been revalidated (Hui & Kottelat, 2009). To date, research pertaining to fish biodiversity in the Batanghari River has predominantly relied on traditional morphological criteria, with limited utilization of comprehensive barcoding techniques. The primary objective of this study is to compile the first DNA barcoding-based inventory of freshwater fish species in the Batanghari River, Jambi, Indonesia. Future studies can use this inventory as a reference for DNA sequence screening. In addition, the genetic diversity of various species of freshwater fish was evaluated. Researchers will be able to use the DNA barcode data produced by this study to monitor and preserve fish diversity in the Batanghari River in particular and Indonesia in general.
Materials and Methods
The sampling was conducted from October to November 2022. The study involved the collection of samples from five prominent watersheds along the Batanghari River, specifically in Muara Teluk Kayu Putih, Batanghari Tebo (Muara Tebo), Batanghari Tabir (Muara Tabir), Batanghari Tembesi (Muara Tembesi), and Batanghari Hilir. These locations were strategically chosen to encompass the entire stretch of the Batanghari River, spanning from its upstream to downstream regions (Fig. 1). Fish were caught using commercial fish gear (gill nets, scoop netting, trapping nets, ground cage nets, trammel nets, fishing nets, and fishing rods) by fishermen. Fish collected were measured in length and weighed, and photos were taken with a camera for further morphological identification. Subsequently, segments of the tail fin were carefully excised from the gathered specimens employing aseptic scissors and tweezers, put in tubes that included 70% ethanol solution for preservation, then transported to the Genomic Laboratory of Nasional Research and Innovation Institute (BRIN, Indonesia), and stored at room temperature until further processing.
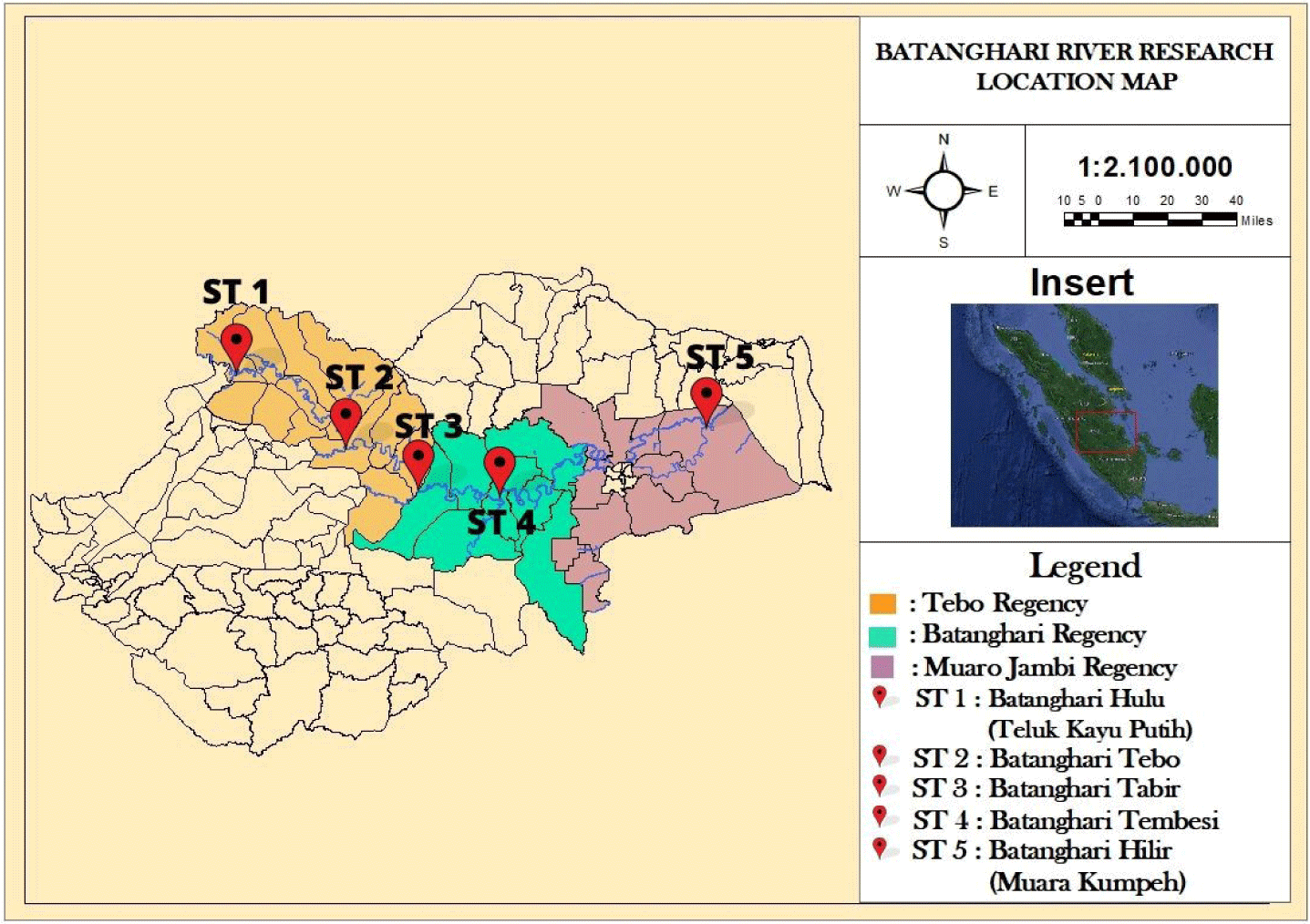
During the surveys, the fish specimens were collected either as live or relatively fresh specimens. The specimens obtained were first identified based on their morphological aspects. For that purpose, each fish specimen was characterized based on the measurement of morphometric characters (head, and body parts). Additional morphological characters, including the snout, mouth, opercle, occiput, fontanel, and eye, morphology of pectoral, dorsal, pelvic, anal, caudal, and adipose (if present) fins, and body shape profile, were also noted and photographed. The measurement of the morphological characters in identifying the species were conducted based on the species identification key and descriptions given by previous ichthyologists for Indonesian fish species (Kottelat, 1993). Additional references from other regions for the same fish species under examination were also used (Rainboth, 1996). When necessary, mainly when the fish species under examination has been taxonomically revised, recent related references were also used. The scientific names of the identified species were referred to the established taxonomy reference (Hui & Kottelat, 2009) species following a catalogue of the fish species in Southeast Asia (Kottelat, 2013) and available information from www.fishbase.org (Froese & Pauly, 2023).
The DNA was extracted from the caudal fin using a DNA extraction kit in accordance with the manufacturer’s protocols (GeneJet Genomic DNA Purification, Thermo Scientific, Waltham, MA, USA). In order to evaluate the efficacy of the genomic DNA extraction procedure, the samples were subjected to mini-horizontal gel electrophoresis. The specimens were introduced into the agarose gel with a concentration of 1.5% (w/v), subjected to an electrical potential of 95 volts (equivalent to 7 volts per centimeter), and allowed to migrate for a duration of 50 minutes. Subsequently, the gel was subjected to staining using 2 μL of 1st Base FloroSafe DNA Stain (First Base Axil Scientific, Singapore). The concentration and purity of DNA were assessed using a NanoDrop™ One/OneC Microvolume UV-Vis Spectrophotometer (Thermo Scientific) and subsequently stored at a temperature of –20°C for subsequent analysis.
The PCR standard was conducted in a final volume of 50 µL using the KOD One ® PCR Master Mix - Blue (Toyobo, Osaka, Japan) commercial kit, following the instructions provided by the manufacturer. A 655-bp fragment of the COI gene was amplified for species barcoding. The amplification was done using the primer pairs COI Fish-F (5'-TTC TCA ACTAACCAYAAAGAYATY GG-3') and COI-FishR (5'-TAGACT TCT GGG TGG CCR AAR AAY CA-3') as described by (Ward et al., 2005). The PCR mixture consisted of 25 μl of master mix, DNA template with a final concentration of 20 ng, and 1.5 μl of each primer with a final concentration of 0.3 μM. The reactions were conducted using a Veriti Thermal Cycler from Thermo Fisher Scientific. The thermal conditions for the experiment were as follows: an initial denaturation at 98°C for 3 minutes, followed by 35 cycles. Each cycle consisted of a denaturation step at 98°C for 10 seconds, an annealing temperature at 60°C for 5 seconds, and an extension at 68°C for 18 seconds. The experiment concluded with a final extension of 5 minutes at 72°C. The PCR products were separated on a 1.5% (w/v) agarose gel. The gel was stained using 1st Base FloroSafe DNA Stain from First Base Axil Scientific and the visualization was done using ultraviolet transillumination. The PCR products that showed satisfactory banding at approximately 655 bp were purified using the QIAquick Gel Extraction Kit from Qiagen. The purified products were then sequenced using the Applied Biosystems 3500 Genetic Analyzer Sequencer, which is manufactured by Thermo Fisher Scientific. The sequencing services were provided by 1st BASE Axil Scientific Pte Ltd in Singapore. The resulting sequences were deposited in the NCBI GenBank genetic database and assigned the following accession numbers: OR357888, OR357890, OR357891, OR357892, OR288567, OR288570, OR288572, OR288574, OR288575, OR288576, OR288578, OQ151816, OQ151817, OQ151819, OQ151820, OQ151821, OQ151822, OQ151826, OQ151827, and OQ151828.
All sequences were aligned and edited in MEGA 11 (Tamura et al., 2021), and manual proofreading. All barcodes acquired were uploaded to GenBank databases. The identification of the COI barcode sequence for each sample was conducted using the BLAST and BOLD databases, with reference to the scientific name or species. The classification of specimens was conducted according to the fish taxonomic systems (Fricke et al., 2022). To determine their conservation status, their information was cross-referenced with the International Union for Conservation of Nature (IUCN) Red List of Threatened Species v. 2022-1 (Table 1). The Kimura two-parameter (K2P) distance (Kimura, 1980) was used to calculate sequence divergences. MEGA software 11 (Tamura et al., 2021) was used to generate an unrooted a neighbor-joining (NJ) tree based on K2P distances. K2P distances were calculated in the following categories: intraspecific distances, interspecific values within the same genus, and interspecific values between different genera within the same family. Using the K2P model and 1,000 bootstrap replications (Felsenstein, 2001), NJ tree was constructed for all COI sequences. MEGA 11 was responsible for both model testing and tree building.
Results
All collected specimens were initially identified using morphological techniques. Morphological identification categorized all specimens (1,692) into 36 species, 30 genera, and 16 families, representing five orders and all fish were identified to the species level (Table 1). In addition, of the identified sample, 58.69% belonged to the order Cypriniformes, Siluriformes (23.46%), and Perciformes (14.78%), respectively (Table 1). As per the 2022 edition of the Red List of Threatened Species published by the IUCN, Chitala lopis has been classified as an extinct species. Furthermore, Tor tambroides has been designated as a species with a declining population. On the other hand, Cyclocheilichthys apogon, Macrochirichthys macrochirus, and Trichopodus trichopterus have been classified as species of least concern (LC), although their populations are also showing a decreasing trend. Furthermore, the other species have been classified as LC and data deficient (DD) (Table 1). The Muara Tebo had the highest percentage of fish abundance and diversity at 48.11%, followed by The Muara Teluk Kayu Putih at 45.52%. The Batanghari Merangin-Tembesi region had a lower percentage of fish abundance and diversity at 14.07%, while Batanghari Hilir (Muara Kumpeh) and Batanghari Tabir had even lower percentages at 12.64% and 6.57%, respectively (Table 2). Morphological characters were conducted by quantifying the dimensions of different anatomical regions (Table S1), and were also noted and photographed, (Fig. S1).
Of 1,692 specimens, we amplified 36 fish samples using PCR. All the sequences formed 650 bp after editing, and no stop codons, insertions, or deletions were found in any of the sequences. The minimum pairwise distance among sequences of observed fish samples based on the K2P was 0.003, and the maximum was 0.331. The average genetic divergence within genera, families, and orders, according to K2P, was 0.05, 0.12, and 0.16, respectively. Genetic distance among families showed a wide range, with the lowest genetic distance of 0.191 obtained between Botiidae and Cyprinidae and between Osphronemidae and Prestolepididae, while Polynemidae and Helostomatidae showed a genetic distance of 0.331 (Fig. 2). Interspecific distance was approximately 2.17 times greater than intraspecific distance.
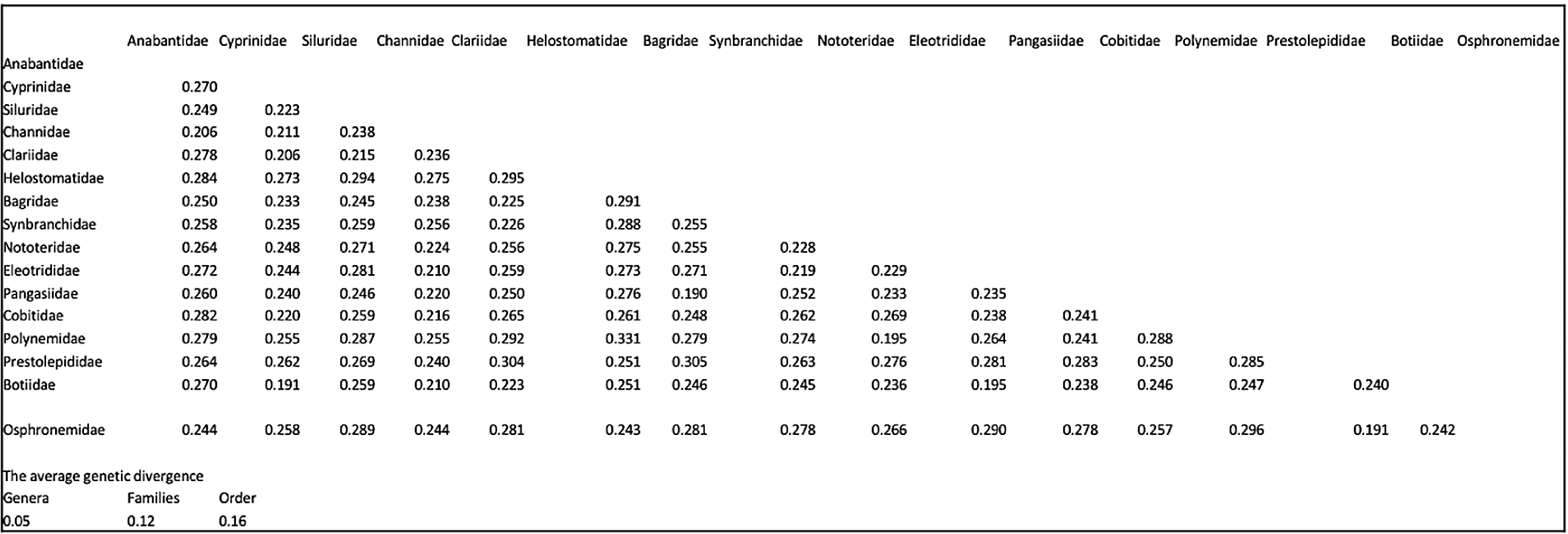
The NJ tree revealed a clear division of the 16 fish families, with all species from the same family forming a monophyletic group (Fig. 3). Also, the phylogenetic tree indicates the presence of three main clades that represent the 16 fish families. The initial clade encompasses species from three families: Cyprinidae, Botidae, and Cobitidae. The second group included species from the families Synbanchidae, Siluridae, and Pangasiidae, while the third clade covered species from the families Osphronemidae, Prestolepididae, Helostomatidae, Anabantidae, Nototeridae, Eleotridae, Channidae, and Polynemidae. The accuracy of all species identifications based on GenBank ranged from 90.24% to 100.00% (Table 3).
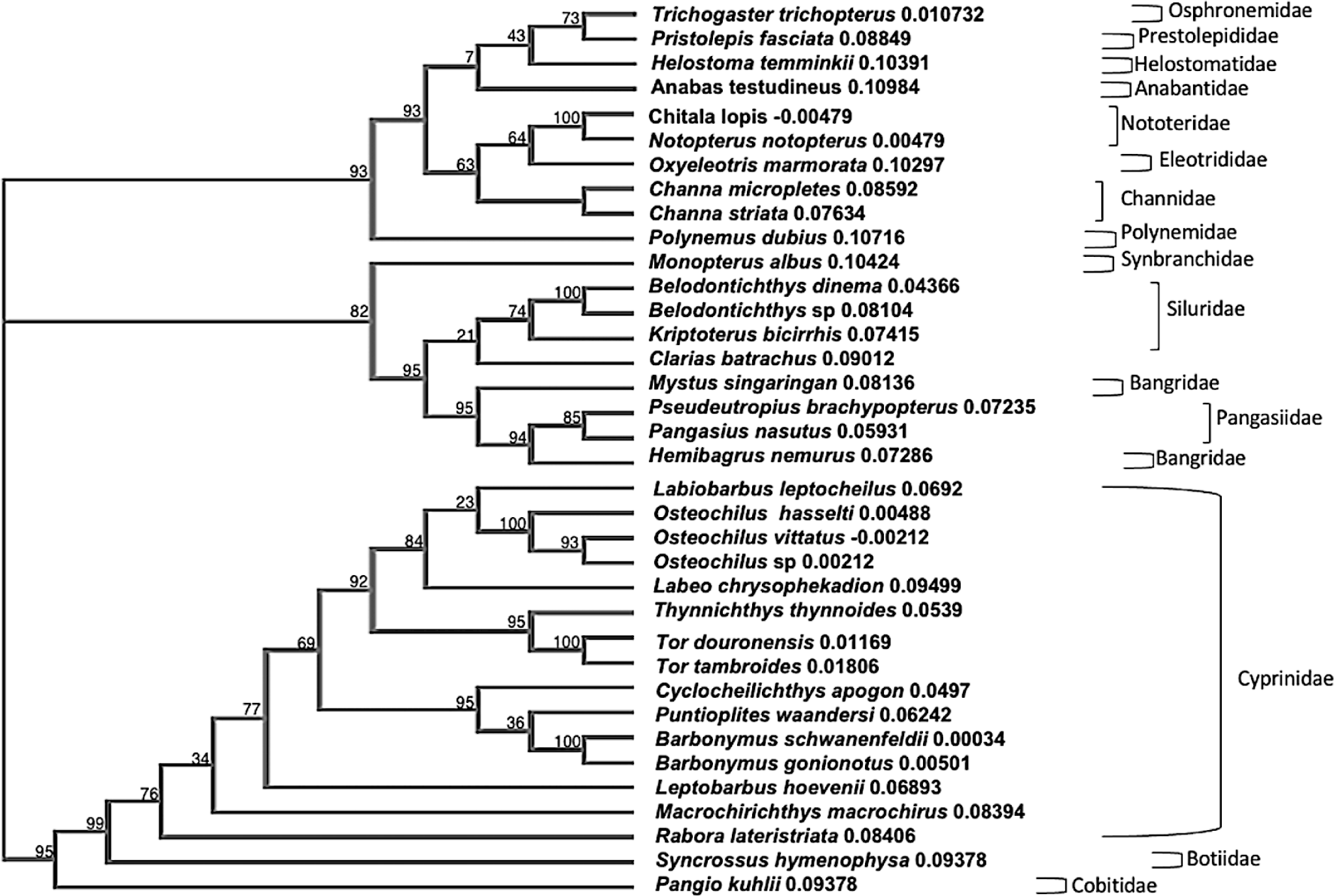
Discussion
A 650-bp-long fragment of the mitochondrial COI gene was used to generate 37 DNA barcodes from 36 species and 16 families. These 16 families represented 25% of the region’s reported fish biodiversity in the Batanghari River (Hui & Kottelat, 2009). This might be because the study was carried out at a number of sampling points, including not only the five major watersheds of the Batanghari River but also all the small rivers and lakes that flow into it. Furthermore, the duration of time required for research to catch fish influences the number of specimens obtained. Moreover, fishing was more challenging when waters were still relatively high in October-November (the wet season), resulting in fewer fish species being caught. It was also indicated that the Batanghari River has been polluted by PETI and industrial waste, as well as by domestic waste (Kaban et al., 2020).
This study clarified the taxonomic status of several taxa, including Osteochilus vittatus and Osteochilus hasseltii, both of which are members of the Osteochilus vittatus group, which is consistent with the findings (Hui & Kottelat, 2009). Another Osteochilus sp. found in Muara Tebo, Batanghari River, is an unnamed species. We identified Pangio semicincta based on morphological analysis, but when we compared it to Pangio kuhli (99.68%) based on COI analysis, Pangio semicincta and Pangio kuhli are both members of the Pangio semicincta group (Kottelat, 2013).
When BLAST searches were used to compare the COI sequences from the 36 species that were gathered, it was found that a lot of them (71.05%) were very similar to GenBank references. However, it is worth noting that a number of the acquired sequences exhibited a lower degree of sequence similarity (ranging from 90% to 96%) when compared to reference sequences sourced from the GenBank database. This includes Channa micropeltes, which displayed a sequence similarity of 95.62%, and Belodontichthys truncatus, which exhibited a sequence similarity of 90.24% (Table 3). The identification of these species may have been limited to the level of genera, as has been demonstrated in several prior investigations (Panprommin et al., 2019). The absence of reference sequences that exhibit complete similarity in the GenBank database leads to their designation as “sp” (Hinchliff & Smith, 2014).
Furthermore, it is worth noting that the mean K2P distance exhibited a higher value among families (0.274) compared to genera (0.256). The observed augmentation in genetic variation corresponded with the escalation in taxonomic hierarchy, suggesting a noticeable divergence in genetic differentiation across genera boundaries. In accordance with the findings of (Sheraliev & Peng, 2021), it was observed that the pairwise genetic distance K2P exhibited an upward trend in relation to the taxonomic hierarchy. Specifically, the values of 0.05, 0.12, and 0.16 were recorded for genera, families, and orders, respectively.
Phylogenetic tree analyses showed a clear relationship between species, by grouping closely related species together in sub-clusters and spacing out distantly related ones. Although one of the goals of barcoding is to identify species boundaries, there is in fact a phylogenetic signal in the data contained in the COI gene. Species of the same genus continued to group together and, in most cases, surpassed the confamilial species cluster (Bariche et al., 2015). In addition, the topology of the NJ tree illustrates species’ relationships (Fig. 3). NJ tree test revealed a clear clustering pattern, providing and informative phylogenetic relationship between taxonomic levels. All fish samples were grouped and separated into distinct clades in accordance with morphological identification and the BLAST algorithm.
The analysis of fish distribution revealed that the upstream segment of the river (Muara Teluk Kayu Putih and Batahnghari Tebo) had greater diversity and species richness, as evidenced by the high values of diversity and richness indices (Tabel 2). The upstream section of the river (Batanghari Tembesi, Batanghari Merangin-Tabir, and Batanghari Hilir) exhibited a comparatively lower degree of human disturbances in contrast to the downstream region, which is characterised by urbanisation and the presence of various pollutants such as industrial waste, domestic waste, and illegal gold mining waste containing mercury. Consequently, the downstream river has become a site of accumulation for these pollutants (Febrianti et al., 2023; Kaban et al., 2020). Moreover, the Batanghari Tebo site demonstrated a significantly greater proportion of fish abundance and diversity in comparison to other locations, which could likely be attributed to the heightened prevalence of macrozoobenthos in this specific area (Febrianti et al., 2023). The preservation of global fish diversity is currently facing significant challenges. In addition to inherent factors that naturally limit species populations, there is an increasing recognition of the detrimental effects caused by introduced species (Radočaj et al., 2021). Concurrently, there is a growing escalation in the detrimental effects of anthropogenic factors on the biodiversity of freshwater rivers (Tickner et al., 2020). The annual decline in the number of biological species has led to the increasing utilization of DNA barcoding as a versatile method for assessing fish biodiversity, observing fish conservation efforts, and managing fisheries (Sheraliev & Peng, 2021). The application of DNA barcoding in our study provides significant benefits to the taxonomic classification of fish species in the Batanghari River. Additionally, it is crucial to address the taxonomic ambiguity surrounding misidentified invasive species that have successfully acclimated to this particular river ecosystem.
Conclusion
The present work revealed the current status of fish diversity in Batanghari River, consisting of 1,692 specimens, 16 families, and 5 orders based on morphological analysis. DNA barcoding COI confirmed that a total of 36 species and 16 families were identified from 36 fish specimens. Our results showed that DNA barcodes using the COI gene sequence was an efficient approach to identify these fish species. This information may be useful for future studies to verify the declining fish diversity issue and managing the fisheries resource for sustainability in Batanghari River.