Introduction
Global, rich in carbonate species lakes are in particular observed in arid and semi-arid environments. As an instance, in East Africa’s Rift Valley, in California around rain-shadowed regions, and in Russia around south Siberia’s Kulunda Steppe, endowed by soda lakes (Sorokin et al., 2014). The phytoplankton of soda lakes inside the remarkable African Rift Valley is usually ruled by cyanobacteria (Luo et al., 2013), especially Arthrospira fusiformis, that is the meals base for masses of heaps of Lesser Flamingos. Even though the practical mono-specific mass boom of Arthrospira is uneven and is, sometimes, substituted via different cyanobacteria or eukaryotic algae (Luo et al., 2013), at the same time as the Arthrospira species is outcompeted, the great life style of benthic diatoms can be perceived (Krienitz & Kotut, 2010; Luo et al., 2013). Because of the reality that numerous benthic diatoms can be used as one greater foods supply to Lesser Flamingos, maximum of the other algae are too small for ingestion by means of the birds (Krienitz & Kotut, 2010; Luo et al., 2013). Among, the soda lake in Ethiopia’s Rift Valley, Lake Chitu is the smallest in size. It is also a deep body of water that remains soda due to soda lakes, which are naturally occurring highly alkaline environments often exhibiting pHs in the 8–12 range (Jones et al., 1998). Rich in carbonate lakes are known for huge amounts of Na2CO3 and Na2CO3 complexes (usually Na2CO3.10H2O or Na2CO3.NaHCO3.2H2O), formed as the result of evaporative concentration under particular conditions of geology, geography, and climate (Jones et al., 1998). They also contain no or very low concentrations of calcium and magnesium ions due to the insolubility of these cations as carbonate minerals under alkaline conditions (Jones et al., 1998). Lake Chitu experiences high evaporative concentration, which is a key factor in its saline-alkaline environment (Ogato & Kifle, 2014).
In soda lakes, for example, an upsurge in salinity has been verified to cause a reduction in biological diversity, notable for the dominance of organisms with high adaptive capability to osmotic stress (Ogato et al., 2015).
Over the past certain years, the Lake Chitu ecology has been considered mono-lake, harboring only Arthrospira spp. (Kebede et al., 1994; Wood & Talling, 1988). Now a day, Lake Chitu is dominated by adaptive microalgae classified under Bacillariophyta and Cyanophyta from the current study report rather than mono-lake characters as reported previously. In comparison to different aquatic ecosystems, soda lake ecosystems are exceptionally touchy to variations in environmental conditions due to herbal or human prompted results (Ogato et al., 2015).
One of the placing records approximately soda a lake is that, no matter reputedly inhospitable caustic situations, those environments are enormously productive. The environmental conditions that can create unique and extreme productive alkaline environments are high buffering capacity, high temperature, light intensity, and an effective unlimited supply of CO2 through the HCO3/CO2 equilibrium (Grant, 2006).
The first studies on Lake Chitu were conducted by Wood & Talling (1988), followed by Kebede et al. (1994). The results reported at that time have also reported shifts in phytoplankton network assemblages related to variations in salinity and alkalinity, mostly due to periodic and inter-annual variations in precipitation and evaporative events (Ogato et al., 2015). Apart from salinity, human-induced disturbances and animal interference have also been shown to affect phytoplankton diversity distribution spatially. Phytoplankton dynamics inside the rich in carbonate species lakes have been studied by means of several researchers who recorded taxa rareness and particular occasions in the temporal existence of various groups of Cyanophyta, Bacillariophyta, and Chlorophyta (Schagerl & Oduor, 2008). Microalgae constitute a distinctly diverse but surprisingly specialized institution of microorganisms tailored to diverse ecological habitats (Severes et al., 2018). Their main habitats are freshwater, brackish, and marine ecosystems. The distribution of phytoplankton community assemblages is inconsistent from place to place. Additionally, Sharma et al. (2013) reported that the correlation of the ecological parameters with the physicochemical parameters of the sampling sites significantly influences the morphophytic diversity of algae in the water bodies. This dynamic of phytoplankton is due to the fact that they respond quickly to environmental changes because of their short life cycle; hereafter, their species composition and dynamics are further expected to indicate the quality of the water in which they are found (Fekadu & Chanie, 2017).
According to the Ogato et al. (2015) report, soda lakes are well represented in Ethiopia and are exemplified by Lake Chitu, which has valuable economic and ecological values. This lake harbors economically important microalgae such as Arthrospira sp. This will be a flock of Flamingos primary food for the hundreds that made the lake appealing, plus a refreshment region, and Arthrospira sp. is likewise used as an alga with excessive nutritional values for people. Accordingly, the phytoplankton community revealed extensive fluctuations whose size needs to be documented to assess the further development of protection and restoration strategies for the lake ecosystem. Consequently, know-how the spatial distribution of phytoplankton urgently had to expand brief-time period and length-term useful resource management techniques, and biodiversity training and training activities are pioneering. The objective of this study concerned into, consequently, to study modifications in phytoplankton network. Additionally, to help narrow the shortage of phytoplankton information on Lake Chitu’s Rift Valley portion and provide o opportunities for monitoring changes in its physicochemical parameters and algae composition.
Materials and Methods
Lake Chitu is one of the soda lakes located 287 km from Addis Ababa and created about 1.5 km south of Lake Shalla at 7°24′0″N and 38°25′0.02″E, within the main Ethiopian Rift Valley Lake. Lake Chitu is found at an altitude of 1,573 m. The lake is a small, comparatively shallow, volcanic crater that is initiated by an explosion when groundwater comes into contact with hot lava or magma (Legesse et al., 2002). The lake is positioned in a depression within a closed basin (Ziway-Shala basin), lacking obvious surface outflows and inflows (Ogato et al., 2015). And also water input by direct precipitation and a few hot springs located at its shores (Ogato et al., 2015). Previously, it was characterized as a natural monoculture of Arthrospira (Ogato et al., 2014), and we know that every day the size of the lake becomes smaller and smaller. This may be due to the lake’s familiarity with high evaporative concentration, which is the major factor in its saline-alkaline nature (Wood & Talling, 1988).
Three representative sampling sites were selected based on human-induced pressures and the topographic nature of the Lake Chitu area (Fig. 1), according to the protocol reported by Etisa et al. (2018). Site one was regarded as a protected area that was covered by forest around the site. Site two was characterized by the impacts of human and animal intervention (anthropogenic areas). Site three was likewise denoted as an inlet, flooding, and bird flock. The study sites were named protected area (PA), anthropogenic areas (AA), and flooding area (FA) (Fig. 1).
Microalgae samples were collected temporally with vials contain 50 mL from selected sites. Temporal sampling of the lakes was made for one cycle in November 2021 and conducted during the November month between 10:00 a.m. and 11:00 a.m. All selected sites, marked with GPS, for the study time. Immediately after sampling, the samples collected from different plots with different factors were mixed in equal proportions to produce composite samples, which were used for enumeration of species composition and abundance. The collected samples were preserved with 4% formaldehyde in 50-mL vials to study the variation of microalgae, as described by Etisa et al. (2018).
A number of in situ physicochemical measures were taken at each selected site, in which electrical conductivity (EC), total dissolved solids (TDS), and surface water temperature were measured on sites using a portable digital multimeter (EC scan 30, AD310, ADWA HUNGARY KFT., Romania). Likewise, pH was measured on sites using a pH meter (pH ep-pocket sized, HACH Instruments, Loveland, CO, USA).
Using samples filtered through Whatman GF/F (pore size 0.45 μm, Sigma-Aldrich, UK), major algal nutrients (NO3– mg/L, NH3 mg/L, NO2-N mg/L and PO4-P mg/L) were determined by the cadmium reduction method, nessler, diazotization method, ascorbic acid, molybdate blue, and alkalinity (CaCO3) meq/L, carbonate (CO3–2) mg/L, bicarbonate (HCO3–) mg/L by titrimetric methods, and fluoride (F–) mg/L by the ion-selective electrode method, respectively (APHA, 1999). Also, some heavy metals such as mercury (Hg) µg/L and arsenic (As) µg/L determined by the hydride AAS method, but silica (SiO2) and chromium (Cr+6) were unfortunately not detected by molybdosilicate and flame AAS, respectively, or were below the detectable capacity of instruments, as reported by APHA (1999, 2012).
After proper collection, water samples were transported to the laboratory to identify the phytoplankton and estimate their abundance; the composite samples were preserved with 4% formalin (recommended for highly alkaline lakes) in 1-L bottles in triplicate. After being preserved with 4% formalin, leave it overnight or for 8 hours for settlement. After settlement, siphon off the half-water sample slowly into the sink, cap the bottle, shake it rigorously, and further transfer it into a 500-mL beaker. After transferring it into a 500-mL beaker, cover the beaker with aluminum foil and dark clothes to prevent contamination and leave it overnight according to Sharma et al. (2015) protocol.
The next day, the settlement was homogenized rigorously and transferred in with the help of a dropper. One drop of sample was transferred onto a microscopic glass slide, placed under a compound microscope, and examined by a 40× magnification lens. Phytoplankton taxa were identified to the species or genus level possible using appropriate keys (e.g., Bellinger & Sigee, 2010; Gasse, 1986; Komárek & Kling, 1991; Komárek et al., 2003), and their relative frequencies were counted in triplicate.
All statistical analysis was done using the statistical program SPSS (Version 20, IBM, Amonk, New York, USA) to determine the temporal variation of physicochemical parameters using one-way analysis of variance (ANOVA). Principal component analysis (PCA) was used to understand the correlation between spatial distribution of phytoplankton groups and physicochemical parameters in Soda Lake Chitu.
Results
The values of physicochemical parameters recorded among the sampling areas during this study are summarized in Tables 1 and 2. Among the physicochemical parameters recorded, ammonia, nitrite, phosphate, PH, and EC had no statistical differences (ANOVA, p < 0.05) at different sampling areas in Lake Chitu. Physicochemical parameters such as carbonate species (carbonate and bicarbonate), alkalinity, nitrate, sulfates, fluoride, and heavy metals (i.e., mercury and arsenic) revealed spatial variations (Tables 1 and 2) at different sampling areas in Lake Chitu.
The mean surface water temperature for the sampling sites ranges from 22.8°C for both PA and AA to 23.2°C for FA. The TDS value for the sampling sites was highest in both PA and AA with 227.7 mg/L while FA recorded the lowest TDS value of 201.0 mg/L. The Arsenic (As µg/L) value shows similar results in all studied sites with 11.1 µg/L. But the value of arsenic (As µg/L) was higher than that of mercury (Hg µg/L) (Fig. 2). The mercury (Hg µg/L) value was highest in PA with 0.32 µg/L, the lowest value was recorded in AA with 0.2 µg/L (Table 2). Previously, no measureable fluoride (F− mg/L) was detected in Lake Chitu. But, currently, the fluoride (F− mg/L) value has been recorded from Lake Chitu for the first time. The mean fluoride (F− mg/L) was highest from AA with a value of 450 mg/L and lowest from FA with a mean of 300 mg/L (Table 1), while the mean CO32– mg/L was highest from AA with a value of 56,100 mg/L and lowest with 4,774 mg/L from PA. Generally, all carbonate and bicarbonate species were low in PA and highest in FA, followed by AA (Fig. 2). The ammonium mg/L value showed no change at all study sites. Also, the value of Nitrate mg/L follows the trends of ammonium (Fig. 2). The amount of sulfate recorded from Lake Chitu was the highest. The highest value of sulfate was recorded in the AA, followed by both the FA and the PA (Fig. 2). At both FA and AA, the value of alkalinity mg/L was higher and relatively low at PA.
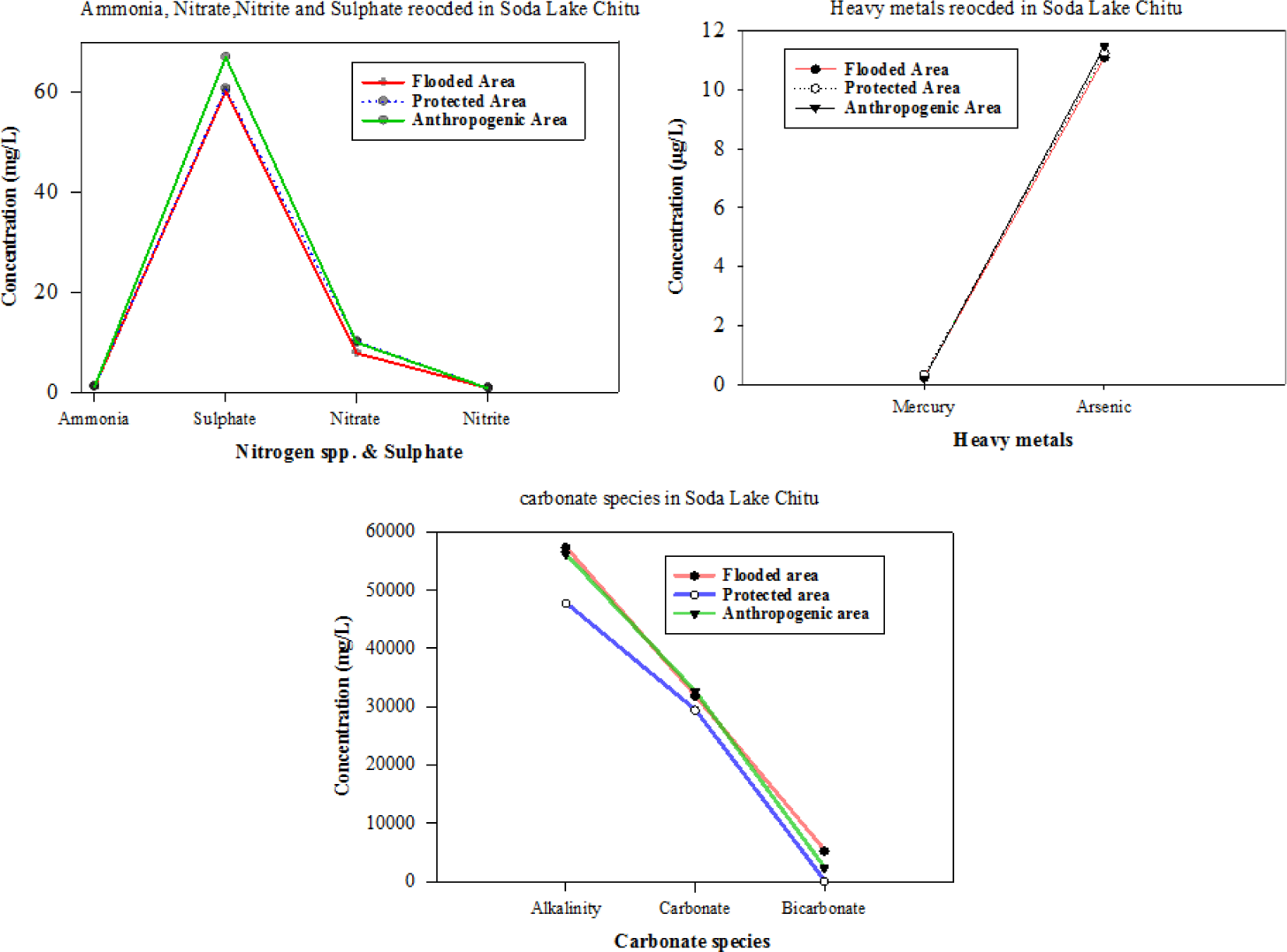
Diversity of microalgae from Lake Chitu: detailed microscopic examination of water samples revealed that a total of 14 species belonging to two phytoplankton phylum, namely Bacillariophyta and Cyanophyta, were identified from the study sites in Lake Chitu (Table 3). The more diverse group was Bacillariophyta (with 8 species), followed by Cyanophyta with 6 species, respectively.
Discussion
Surface water temperature (°C) and TDS (mg/L) are among the most significant physical factors affecting the distribution and temporal variations of phytoplankton (Fekadu & Chanie, 2017). In the present study, Lake Chitu’s surface water temperature varied between 22.8 ± 0.07°C to 23.2 ± 0.12°C during the sampling time of the day. The pH values in this study varied from 9.9 to 9.97. A lower pH (9.9) was measured at the site FA, whereas the highest (9.97) was recorded at the site PA. The reason why pH values of Rift Valley Lakes become high is due to the natural weathering process of the surrounding alkaline volcanic rocks and the tectonic geological background (Kebede et al., 1994; Lameck et al., 2023).
The current nitrate (9,126.7 µg/L) values exceed in thousands the formerly reported values for the same lake (1,700 µg/L, Wood & Talling, 1988; 20 µg/L, Ogato et al., 2014), indicating a eutrophication trend with time.
The sulfate ion (1.305 mill equivalents (meq/L) recorded currently in Lake Chitu was extremely lower than the value previously recorded (17.7 meq/L) by Wood & Talling, 1988, among the anions in Lake Chitu. Lameck et al. (2023) elaborated that the microbial reduction of sulfate ions to sulfide through organic matter degradation and the loss of sulfate by sorption on the surface are explanations for the sulfate ion content in Rift Valley Soda Lakes.
Previously, the depletion of SiO2 and remarkable disappearance of diatoms were reported by Ogato et al. (2015); in contrast, the abundance of diatoms was high during the current study. Therefore, the depletion of SiO2 can be associated with the abundance of diatoms. The non-detectability of silica (SiO2) did not affect the abundance and diversity of Bacillariophyta (diatom) in Lake Chitu during the study time. Biological diversity and community structure, especially in soda lakes, seem to be controlled by salinity and the degree of environmental stability (Schagerl & Oduor, 2008).
The heavy metal species (mercury and arsenic) were measured in the study lake for the first time. The value of mercury (11.3 µg/L) recorded was relatively higher than the allowable concentration (µg/L). According to the study by the B.C. Ministry of Environment and Climate Change Strategy (2021), to protect freshwater aquatic life from the acute effects of mercury, the maximum concentration of total mercury in water should not exceed 0.1 µg/L. But currently, the value recorded from Lake Chitu exceeds 100 times the above-reported value. Heavy metals arise in small quantities naturally and may come into aquatic systems through the percolating of rocks, aerial dust, forest fires, and vegetation (Ogoyi et al., 2011).
Heavy metals cannot be degraded; they are persistently placed and present in water, sediment, and aquatic organisms, consequently causing heavy metal pollution in water bodies (Ogoyi et al., 2011). The occurrence of heavy metals in the water may have a thoughtful effect on the microalgae, which establish the main food basis for bivalve mollusks in all their growth phases, zooplankton (rotifers, copepods, and brine shrimps), and larval periods of certain crustacean and fish species (Ogoyi et al., 2011).
The conductivity value recorded in the current study (398 mS/cm) extremely less than previously recorded from the same lakes (28,600 mS/cm, Wood & Talling, 1988; 56,330 mS/cm, Ogato et al., 2014). Alkalinity (53,720 mg/L) relatively recorded in this study and was far higher than the values reported by (4.46 meq/L or 2,230 mg/L Wood & Talling, 1988); 36.8 g/L as CaCO3 (36,800 mg/L) Ogato et al. (2014). The relatively high values of EC observed are due to the evaporation of water, which leaves a higher concentration of salt within a smaller volume of water (Idowu & Ugwumba, 2005). The recorded high levels of carbonate-bicarbonate alkalinity (as mg/L CaCO3), pH, salinity, conductivity, Na+, Cl−, and PO4-P in Lake Chitu are characteristic of soda lakes (Ogato et al., 2014). Most of the currently recorded chemical parameters were considerably lower in Lake Chitu than previously recorded by Ogato et al. (2014) in Lake Shala (Table 2).
The existence of high alkaline-sodic structures in soda lakes may be due to an unbalanced preliminary stage of carbonate alkalinity and calcium [(HCO3–) > 2(Ca2+ + Mg2+)], initiated by the weathering and dissolution of volcanic rocks (Lameck et al., 2023). In Ethiopian Rift Valley Lakes, most of the alkalinity derives from the hydrolysis of volcanic glass and lava and the occurrence of high solar radiation, moderate temperature, limitless carbon dioxide reserves in the form of carbonate-bicarbonate salts, and other essential nutrients such as phosphate. The East African soda lakes (EASL) have high amounts of photosynthesis; this system forms high concentrations of Na+ and HCO3– in the dilute inflow of Rift Valley Lakes (Grant, 2006; Kebede et al., 1994; Schagerl & Oduor, 2008; Seyoum, 2016).
The main mechanisms for the formation of these ions are the leaching of rock materials rich in Na from volcanic rocks in the catchment areas and evaporative concentration enhanced by the dry and warm climate (Ogato et al., 2014). In most saline lakes in arid regions, these dominant ions are responsible for the high pH, alkalinity, conductivity, and salinity of their waters (Kebede et al., 1994; Wood & Talling, 1988).
The SO2 values of Lake Chitu (60.2 ± 0.012 to 67.03 ± 0.56 mg/L) were higher than the value recorded from Lake Hawassa 35 mg/L (Abate et al., 2015; Kebede et al., 1994). But this value is far less than the previously recorded values from the same lake: Lake Chitu reported a value of 1012.8 mg/L (Huib & Herco, 2006; Kebede et al., 1994). This was mainly attributed to the high rate of evaporation and consequent reduction in the water level during the spring.
The alkaline nature of Lake Chitu along its shore explains these ion dominance patterns (Lameck et al., 2023). The dissolution of alkaline rocks explains the dominance of Na+ and K+ in Lake Chitu. In the case of the dominance of monovalent elements over divalent elements, Na+ is in higher abundance compared to K+. The enhancement of Na+ over K+ is well-known because Na+ is a conservative species as its concentrations remain constant during the evaporation process, while K+ can be removed by the adsorption process on the active surface of the lake (Lameck et al., 2023). The primary precipitation of calcite and dolomite minerals results for the lower contribution of Ca2+ and Mg2+ to the total cations of Lake Chitu, which is true from the review of inland water and soda lake by Getenet et al. (2022, 2023); Lameck et al. (2023).
The higher dominance of the Cl– ions can be initiated by the high precipitation rate and trona input of bicarbonate depletion and chloride enrichment in brines, which occur in conjunction with an increase in the chlorinity of Lake Chitu. The conservative nature of the Cl– over the higher range causes it to persist in the solutions even beneath high evaporation rates (Lameck et al., 2023). The nearby hot spring’s water concentrated by evaporation discharges water into Lake Chitu; alkaline hot springs recharge; and dissolution of host volcanic bedrock contribute to the higher dominance of Cl– in Lake Chitu.
Two major phytoplankton phyla, namely Bacillariophyta (diatom) and Cyanophyta, were identified from Lake Chitu. Lake Chitu had the smallest phytoplankton species richness compared to the other two nearby lakes, such as Lake Shall and Abijatta. This paper showed the phytoplankton diversity in terms of frequency and spatial aspects, along with some physicochemical parameters, in Lake Chitu, Ethiopia.
In addition to the phytoplankton diversity issues described in the literature concerning this topic, this research presents a spatial element by classifying the factor types, judging their current distribution and extent, and correlating them with some physicochemical parameters measured during the study time.
Our recent study of the spatial variation of phytoplankton with environmental factors is unsatisfactory in Lake Chitu. Spatial variations in phytoplankton are related to some environmental factors in aquatic environments (Fekadu & Chanie, 2017). Also, the phytoplankton community of Lake Chitu is comprised of taxa characteristic of soda-alkaline lakes (Ogato & Kifle, 2017), including the species typically inhabiting tropical soda lakes (e.g., Arthrospira). The recorded species number is indicative of the occurrence of a relatively two-phylum phytoplankton community, mainly Bacillariophyta and Cyanophyta, similar to Arthrospira-dominated soda lakes in East Africa, including Lakes Nakuru and Bogoria in Kenya (Ballot et al., 2004; Kaggwa et al., 2013; Okoth et al., 2009) and Lake Chitu in Ethiopia (Kebede et al., 1994).
The phytoplankton community was dominated by Bacillariophyta (diatom) in terms of species richness with Navicula sp., Navicula cryptocephala, Nitzschia gracilis and Eunotia sp. having most of the species. The Cyanophyta (M. aeruginosa, S. platensis/fusiformis, and Chroococcus minutus) were the most abundant microalgae in Lake Chitu. M. aeruginosa dominated the microalgae diversity at all sampling locations (PA, AA, and FA) (Table 3).
Bacillariophyta (diatom) contributed more in terms of frequency of occurrence than Cyanophyta (Table 4). Bacillariophyta (diatom) were represented by 8 genera and blue-green algae by 6 genera (Table 4). The most common Bacillariophyta (diatom) throughout the study period were represented by Navicula spp., N. cryptocephala, N. gracilis, and Eunotia sp. Cyanophyta were very commonly represented by M. aeruginosa, S. platensis/fusiformis, and C. minutus. Out of the 14 phytoplankton identified, 5 species were restricted to the PA location; only two, Epithemia sp. and Fragilaria capucina, were recorded from the FA, while 7 species occurred at the AA (Table 3).
The distribution trends showed a high similarity of phytoplankton species and a small spatial difference in abundance at each study area (Fig. 3) except at PA species become varies in distribution. Bacillariophyta accounted for most species of phytoplankton in the investigated waters, both in richness and frequency. Navicula spp., N. cryptocephala, N. gracilis, and Eunotia sp. were identified as dominant species based on the percent calculated, which contributed 57.1% of the total number of phytoplankton.
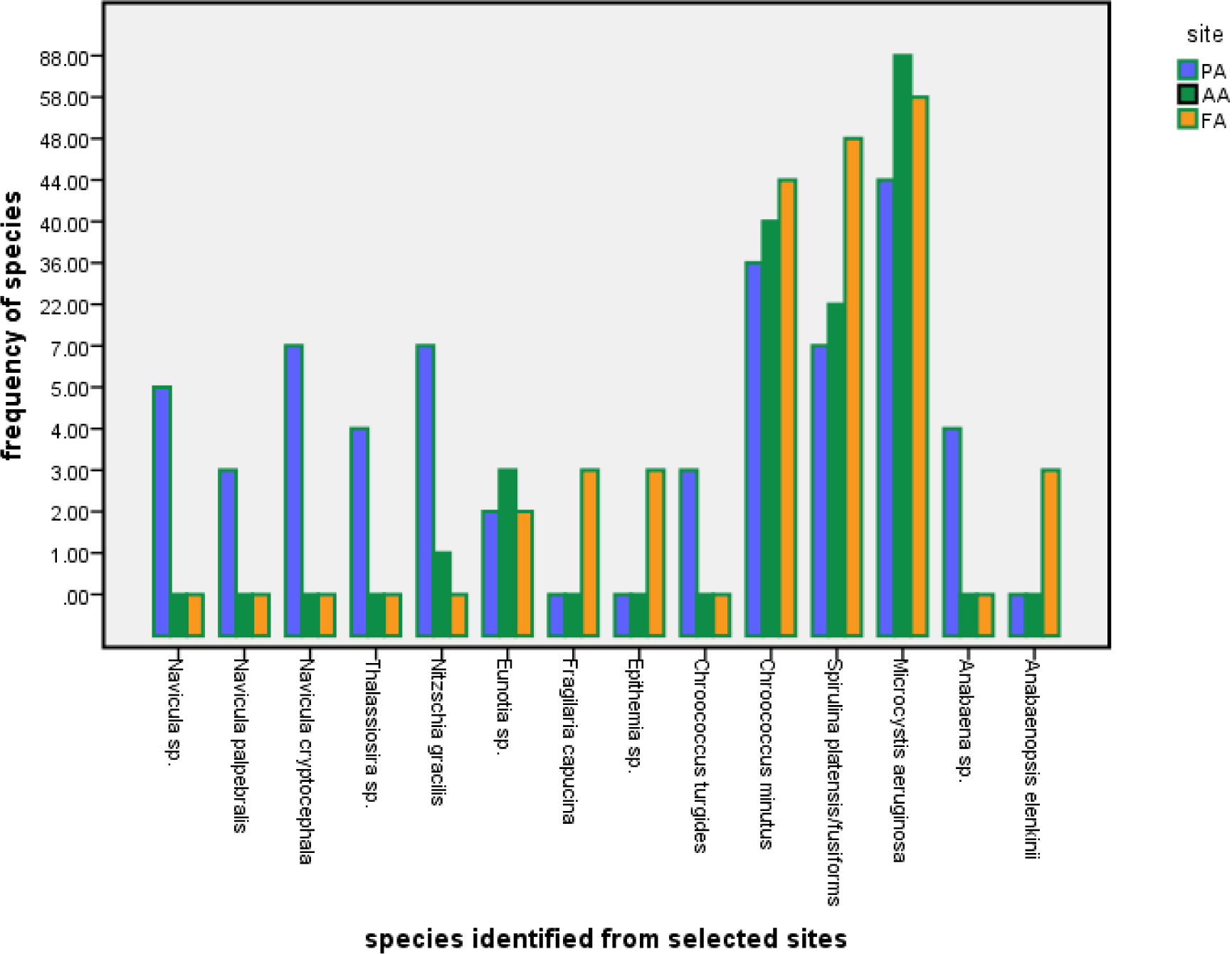
When comparing the current phytoplankton community species composition in Lake Chitu with previous records, there is a little bit of agreement in phytoplankton communities, with most species changes in long-term species numbers over the past decades. A number of species, mostly of the phylum Bacillariophyta (Epithemia zebra, Navicula halophila, Cyclotella Spp., and Rhopalodia Spp.) and Cyanophyta (only Arthrospira fusiformis), have already been reported by Kumssa & Bekele (2014).
Also, earlier studied reports by Ogato et al. (2015) indicated additionally that from the phylum Bacillariophyta, Rhopalodia gibba (Ehr.) O. Muller, R. gibberula (Ehr.) O. Muller and Rhopalodia sp. O. Muller’s Synedra sp. were not recorded recently. Also, no Cryptomonad species were observed in the current study. Earlier studies by Ogato et al. (2015) reported similar results from both Cyanophyta (S. platensis/fusiformis, Anabaena sp.) and Bacillariophyta (Navicula spp., Nitzschia spp.). From the spatial frequency distribution (Figs. 4 and 5), at all study sites, Cyanophyta taxa were relatively dominant and highly represented by both M. aeruginosa, S. platensis/fusiformis, and C. minutus. The dominance of the above Cyanophyta phylum may be due to elevated temperature, high pH, may be they excrete a compound which suppresses the growth of competing algae, and the capacity to float and form scums (Fenta & Kidanemariam, 2016).
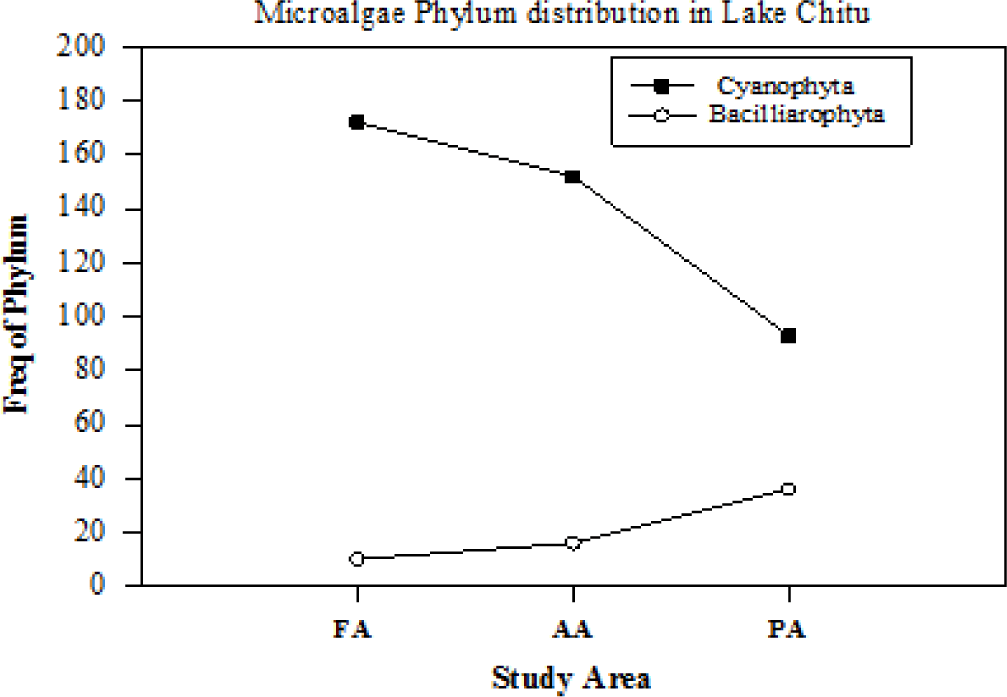
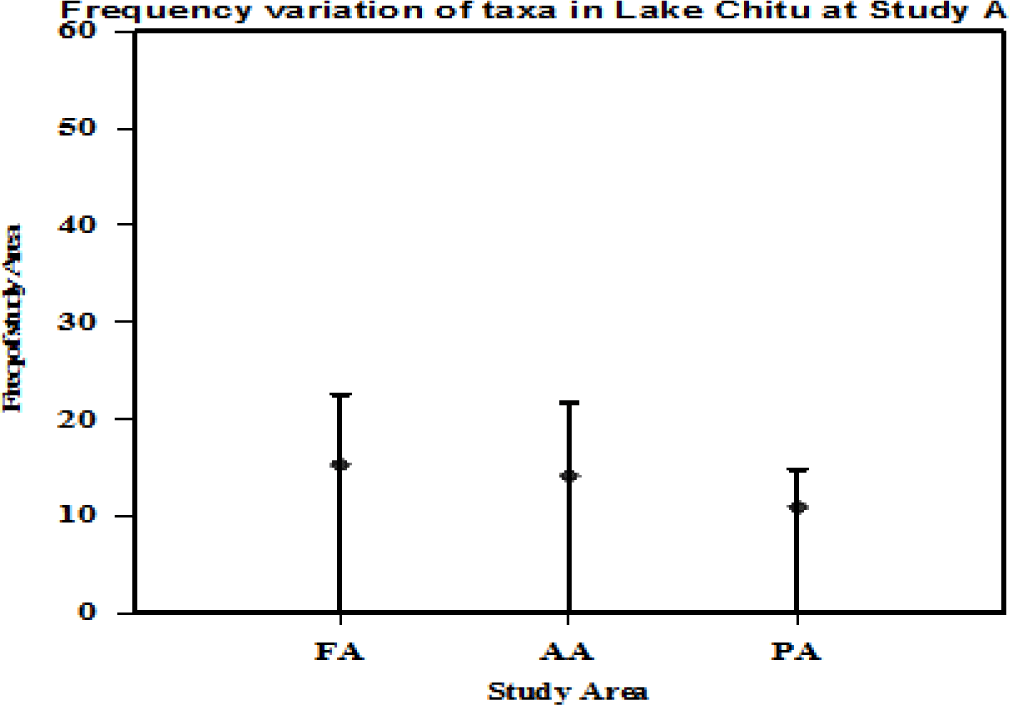
Microalgae variations observed in this Lake Chitu was slightly an expression of both human-induced and natural characteristics of physiological stress to which the phytoplanktons were subjected in the presence of high carbonate species such as alkalinity (CaCO3), carbonate (CO32–), and bicarbonate (HCO3–), moderate inorganic nutrients, and some heavy metals. Some heavy metals, such as silica (SiO2) and chromium (Cr+6) were undetectable or otherwise very small, with almost uniform distribution throughout the water column.
To determine the relationship between phytoplankton community composition and the stations in the waters of Lake Chitu, we used PCA. Based on the results of the analysis using PCA and the root value (eigen value), most of the phytoplankton communities recorded were loaded around the principal component (PCA, 1), which accounts for 89.24% (Fig. 6). The cumulative value of variance (loading factors) and the correlation matrix showed that M. aeruginosa and C. minutus were situated and loaded almost all around the principal component (PCA, 1). Additionally, S. platensis/fusiformis loading is determined by the FA. The other taxa are situated around the center of the axis and determined by both AA and PA. The proximity of a station based on related phytoplankton community composition (Fig. 6), where adjacent stations have the same value of these phytoplankton community compositions. Station AA close to (PCA, 1) with phytoplankton community compositions that determined them are, negative relationship between the right-sided species (e.g. C. minutus and M. aeruginosa) and the left-sided species (e.g. Anabaenopsis elenkinii, N. gracilis, Thalassiosira sp. and Eunotia sp.). However, (PCA, 2) has a fairly low relationship with phytoplankton community compositions.
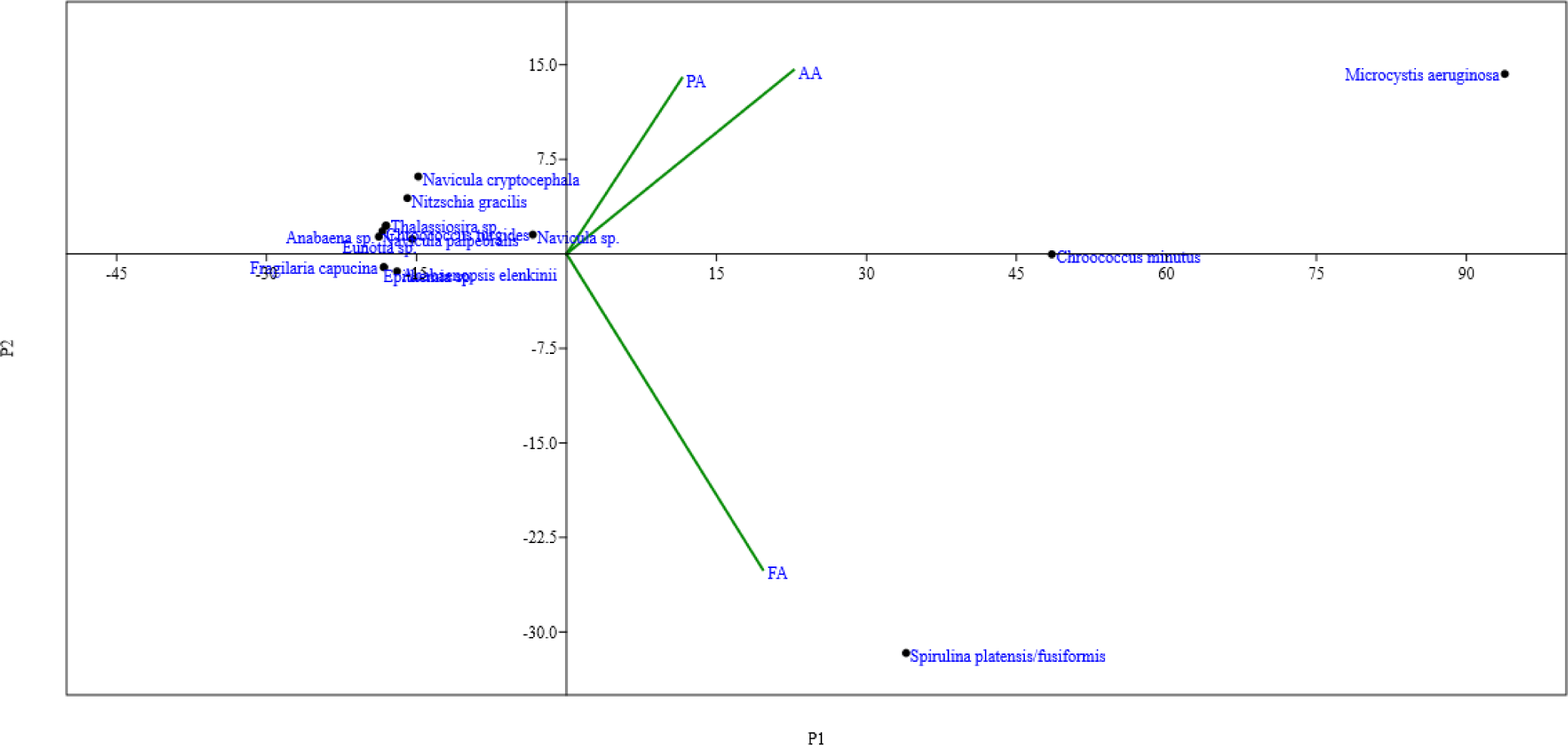
Conclusion
Our study focuses on the ecologically and economically valuable phytoplankton community changes and composition in Lake Chitu, which represents Lake Chitu phytoplankton distribution spatially. Two major phytoplankton phyla, namely Bacillariophyta (diatom) and Cyanophyta, were identified in Lake Chitu. The phytoplankton diversity found in this study is mostly distributed in several Rift Valleys in Ethiopia. Almost all study sites harbor predominantly M. aeruginosa, Chroococcus turgides, and S. platensis/fusiformis. Our result shows that the responses of most physicochemical parameters are important factors shaping phytoplankton community changes in Soda Lake Chitu, Ethiopia. The highest sulfate, carbonate species, alkalinity, and heavy metal (arsenic) values were recorded. Lake Chitu, with a high pH value and carbonate content, is characterized by the dominance of pennate diatoms in the phytoplankton. Lake Chitu currently requires a high degree of attention from stakeholder groups, policymakers, scientists, and the government due to the fact that the lake harbors applicable species in several economic sectors, such as S. platensis/Fusiformis, which act as one of the most valuable e economic resources of the lake ecosystems. This makes Lake Chitu a future hot spot area to harvest S. platensis/Fusiformis species and recreational site.