Background
Phosphorus (P) is a component of organic phosphate in diverse forms, such as nucleotides, phospholipids, coenzymes, deoxyribonucleic acid, and ribonucleic acid. Inorganic phosphates also serve as important buffers to maintain normal pH of intra- and extra-cellular fluids (Zubay 1983). Fish have the dietary requirement of P like other animals for growth. The requirement was reported to range from 0.3 to 0.6% for channel catfish (Wilson et al. 1982), rainbow trout (Rodehutscord and Pfeffer 1995), striped bass (Brown et al. 1993; Dougall et al. 1996), and white fish (Vilema et al. 2002). However, somewhat higher requirement value of 0.7 to 0.9% was estimated for haddock (Roy and Lall 2003), red tilapia (Phromkunthong and Udom 2008), African catfish (Nwanna et al. 2009), red drum (Davis and Robinson 1987), yellow croaker (Ma et al. 2006), and carp (Kim et al. 1998). On the other hand, P discharged into water stream as a major nutrient for eutrophication is known to play a vital role in promoting algal growth (Beveridge 1984; Auer et al. 1986).
Theoretically, diet for fish should contain all essential nutrients including P above the needs and maintain total P levels as low as its available requirements in order to achieve both maximal growth and minimum P discharge. However, the practical diet contains an excessive P originating from animal and plant sources, which is excreted to the cultural water body due to the low availability by stomachless species like carp (Kim and Ahn 1993). Therefore, soluble P sources like monocalcium phosphate (MCP) and dicalcium phosphate (DCP) are being supplemented to the diet to meet the requirement of P for maximum growth. Based on this point of views, some significant discharge of P is inevitable from fish farming. Kim et al. (1998) reported dietary available P of 0.7% with 2% MCP exerted both maximal growth and minimum P loss in juvenile carp. An adequate combination of low-P protein meals supplemented with 0.5% MCP significantly reduced the P loading on rainbow trout without compromising the growth (Satoh et al. 2003; Hernandez et al. 2004, 2005).
Such an important source for living creatures as one of non-renewable elements in the nature is mostly produced from phosphate rock of which production in the world has constantly increased from 198 million metric tons in 2011 to 210 million metric tons in 2012 according to U.S. Geological Survey (USGS 2013). Shu et al. (2006) expected all reserves of phosphate rock would be depleted by 2090, assuming an annual increase of 1.5% in its demand. Considering this estimation, it is necessary to recover phosphorous from diverse downstream of agricultural and industrial fields. One of resources to retrieve phosphorous could be swine manure which contains high levels of phosphorous and nitrogen. Swine manure becomes a source of pollution on surface waters and induces eutrophication near the site when it is under improper treatment. Thus, the control of wastewater stream must be achieved and struvite precipitation could be an effective way to control phosphorus from wastewater stream with the addition of magnesium (Liu et al. 2011). If this alternative phosphate recovered from swine manure could be effectively employed as an available P source for animals including fish, it would not only substitute for import of several phosphates but also protect our environment through recycling of the waste source.
Cyprinid is the species the most cultured worldwide. In 2012, China produced more than 90% of the world’s carp which relies on formulated feed with the exception of filter-feeding species (Cao et al. 2015). Considering the low availability of P in both animal and plant feedstuffs for carp (NRC 1983), the amount of dietary P supplemented through its additive would be substantial. Nevertheless, the potential use of dietary alternative phosphate as an available P source for growth of the fish was not examined until now. This study was therefore carried out to investigate the supplemental effect of various conventional phosphate sources and the alternative (MHP) on growth, feed utilization, plasma inorganic P, whole body P, and the availability of juvenile carp.
Methods
The protocol for the present experiment was approved by the Institutional Animal Care and Use Committee of Kangwon National University, Chuncheon, Republic of Korea. Commercially selling phosphate additives (MCP, BIOFOS®, USA; DCP, SICHUAN MIANJHUSANJIA FEED Co., China; tricalcium phosphate (TCP), FOODCHEM, Shandong, China) were obtained from fish feed companies in Korea, and the testing P additive, magnesium hydrogen phosphate (MHP, MgHPO4), was prepared from P-recovery process in swine farm operated by Kangwon National University.
A P-recovery process was a pilot scale with the effective volume of 400 L, and the operational condition was set with hydraulic retention time of 3 h and pH 8 to 9 controlled by aeration (33 L air/m3reactor.min) resulting in CO2 stripping. Such a condition leads to precipitate crystal called struvite which is composed of magnesium, ammonium, and phosphate in equimolar ratio. In order to provide magnesium source that is a deficient source in swine manure, magnesium chloride (MgCl2) was added to meet Mg to P ratio of approximately 1.2.
The precipitates collected was struvite, which was identified by X-ray diffractometer (XRD) (Rigaku, Model D/Max-2500 V, Japan). The MHP was obtained by removing ammonium-N through incineration of the recovered struvite at 550 °C for 30 min. It was finely ground to use as phosphate additive.
The diet was prepared with MCP, DCP, TCP, and MHP at the level of 2% but the control diet did not contain phosphate source of 2% in lieu of cellulose. The major ingredients were constituted with fish meal (25%), soybean meal (40%), wheat flour (27%), fish oil (2%), and soy oil (2%) as a basal diet containing 42.5% protein and 6.5% lipid (Table 1). Prior to diet formulation, chemical composition of fish meal, soybean meal, wheat flour, and four phosphate sources were determined. All the ingredients were weighed following the formula and ground to 100-mesh size by a hammer mill and thoroughly mixed for 10 min using a V-mixer (Hangjin Co., Korea) in order to make a mixture of 500 kg per diet. Then, the mixture was transferred to a twin extruder (Model ATX-2, Fesco Precision Co., Korea) and manufactured to the sinking pellets with two sizes of 1.5 and 2.5 mm, respectively, for feeding during the first 6- and second 3-week growth trials, respectively. Operating conditions of extrusion were as follows: feeder speed, 16~18 rpm; conditioner temperature, 80~90 °C; main screw speed, 250~320 rpm; temperature of the second and the third barrel compartment, 105~135 °C; steam heater pressure, 4~6 kgf/cm2 and temperature of the fourth barrel compartment, 80~90 °C. Extruded pellets were dried at 60 °C for 6 h using a drying oven which resulted in the moderate moisture content of 5 to 8%.
MCP, monocalcium phosphate, DCP dicalcium phosphate, TCP tricalcium phosphate, MHP magnesium hydrogen phosphate
aVitamin added to supply the following (per kg diet): vitamin A, 4000 IU; vitamin D3, 800 IU; vitamin E, 150 IU; vitamin K3, 20 mg; thiamine HCl, 25 mg; riboflavin, 50 mg; D-Ca pantothenate, 100 mg; biotin, 1 mg; folic acid, 20 mg; vitamin B12, 0.2 mg; niacin, 200 mg; pyridoxine HCl, 20 mg; ascorbic acid, 500 mg; inositol, 200 mg; BHT, 15 mg; BHA, 15 mg
bMineral added to supply the following (per kg diet): copper sulfate (25.4% Cu), 30.5 mg; zinc sulfate (22.7% Zn), 230 mg; manganous sulfate (32.5% Mn), 100 mg; cobalt chloride (24.8% Co), 20 mg; potassium iodide (76.4% I), 6.5 mg; sodium selenite (45.6% Se), 2.2 mg; sodium fluoride (45.2% F), 8 mg
cAvailable P calculated based on apparent digestibility coefficient values
For the measurement of P digestibility, chromic oxide of 1.0% was mixed with an aliquot of 10 kg of each extruded diet fully ground. Then, each diet mixture was added with 20% distilled water, and the mixture was pelletized using a meat chopper and dried for 12 h in a ventilated oven at 60 °C. The diets were stored in a freezer at −20 °C for P digestibility measurement until the growth trials.
Carp fry of 5000 with around body weight of 5 g were kindly provided from Kyeongnam freshwater fish institute and acclimated to the experimental conditions for 3 weeks with a control diet. Following a 24-h fasting, five groups (triplicates/group) of 450 fish of a mean body weight of 6.6 g were randomly allotted to each of 15 tanks (0.4 × 0.6 × 0.36 cm, water volume of 66 L). The feeding experiment lasted 9 weeks during which each diet was hand-fed to apparent satiety twice a day (08:30 and 17:30) at the 4% of body weight in every 6 days per week. A recirculation freshwater system was employed where water temperatures for first, second, and third weeks were maintained at 26 ± 1.2, 22 ± 1.2, and 18 ± 1.5 °C, respectively, and dissolved oxygen 5.5~6.4 mg O2/L for the whole experimental periods. The flow rate of 5 L/min was constantly set. The extruded pellet of 2.5 mm size was fed for the last feeding of 3 weeks. Fish were bulk-weighed at the beginning of the experiment in every 3 weeks. Daily feed intake (DFI, %/av. body weight/d), weight gain (WG, %), feed conversion ratio (FCR), protein efficiency ratio (PER), specific growth rate (SGR, %), and survival rate (SR, %) were calculated as follows:
and
At the end of the growth trial, digestibility measurement was conducted to calculate the available P of the experimental diets. Following a 24-h fasting, 300 fish (mean body weight, 22.2 g) were randomly distributed into each of five 130 L capacity tanks (60 fish/tank) with a fecal collection column. Following 1 week of feeding, fecal collections were made for three consecutive weeks as described by Kim et al. (2006). Each diet was fed by hand to apparent satiety twice a day (08:30 and 16:30). One hour after final feeding of the day, the drain pipes and fecal collection columns were thoroughly cleaned with a brush to remove feed residues and feces from the system. The settled feces and surrounding water were carefully collected into 250-ml centrifuge bottles each morning (08:00). Apparent digestibility coefficient (ADC) of P in experimental diets was calculated according to Maynard and Loosli (1969). P availability of phosphate additives was calculated by dividing the difference of total P into that of available P between control and each phosphate containing diet:
where ID is % indicator in the diet, PF represents % P in the feces, IF indicates % indicator in the feces, and PD is % P in the diet.
P availability of the phosphorus additives was calculated according to the following equation:
where APDP indicates % available P in the diet containing P source, APCD is % available P in control diet, TPDP shows % total P in the diet containing P source, and TPCD is % total P in control diet.
At the end of the experimental period, fish were anesthetized with AQUI-S (New Zealand Ltd., Lower Hutt, NZ) and bulk-weighed and counted for calculation of WG, FCR, SGR, PER, and SR. Blood samples were obtained from the caudal vessels with a heparinized syringe from two fish of each tank after fish were starved for 24 h and anesthetized with AQUI-S. Feces collected in the same bottle from each tank for 6 days a week were used as one replicate for the treatment. After collection of three replicate samples of each diet during 3 weeks, fecal samples were lyophilized, finely ground, and frozen at −20 °C until analysis.
Chemical analyses of feed ingredient, diets, and feces were performed by the standard procedure of AOAC (1990) for moisture, crude protein, crude fat, and crude ash. Moisture content was obtained after drying in an oven at 105 °C for 24 h. Crude protein (N × 6.25) was determined by Kjeldahl method after acid digestion. Crude fat was determined by the soxhlet extraction method by using Soxtec system 1046 (Foss, Hoganas, Sweden) and crude ash from incineration in a muffle furnace at 550 °C for 12 h. Chromium in diets and feces for P digestibility measurement was analyzed using a spectrophotometer (Shimadzu, UV-120-12) at a wavelength of 440 nm after perchloric acid digestion (Bolin et al. 1952). Ca in diets and P in diets and the whole body of the final fish (five fish per replicate) were measured using inductively coupled plasma mass spectrometer (ICP-MS) (Perkin-Elmer, NexION 300D, Waltham, MA, USA) after the pretreatment of test materials following the method from US Environmental protection agency (USEPA 1996). Hematocrit (PCV, %) and hemoglobin (Hb, g/dL) were measured with the same fish (two fish per replicate) by the microhematocrit method (Brown 1980) and the cyanmethemoglobin procedure using Drabkin’s solution, respectively. Hb standard prepared from human blood (Sigma Chemical, St. Louis, MO) was employed. Blood plasma from two fish per replicate was obtained after blood centrifugation (3500×g, 5 min, 4 °C) and stored at −80 °C until inorganic P (Pi) was analyzed. The plasma Pi was measured using a blood chemical analyzer (HITACHI 7600-210, Hitachi High-Technologies Co., Ltd., Japan) with commercial clinical investigation reagent (Clinimate IP, Sekisui medical Co., Ltd., Tokyo, Japan).
Data of growth trial (initial and final fish, DFI, WG, FE, PER, SGR, and SR), P availability among both replications and treatments, whole body P, and hematological and serological parameters were analyzed using one-way analysis of variance (ANOVA), and significant differences among treatment means were compared using Duncan’s multiple range test (Duncan 1955). Prior to the analysis, homogeneity of variance of all data was verified using Cochran’s test (Sokal and Rohlf 1994). All statistical analyses were carried out using the SPSS Version 10 (SPSS 1999). Statistical significance of the differences was determined by a significant level of 5% (p < 0.05).
Results
As shown in Table 1, crude protein level of dry diets was determined to be 42.2 (DCP) to 42.9% (MCP) and crude fat to be 6.6 (DCP) to 6.9% (control). At the end of the 9-week growth trial, daily feed intake (DFI, %) per averaged weight of fish ranged from 1.88 (MHP) to 2.17 (control and TCP). Fish fed the MHP showed the highest WG of 278%, which was not significantly different (p > 0.05) from that (270%) of fish fed the MCP, while fish fed the control and TCP showed lowest WG among the treatment groups (p < 0.05). FCR ranged from 0.99 (MHP) to 1.29 (control). The highest value of PER and SGR were found in fish fed the MCP and MHP, while fish fed the control and TCP showed the lowest level (Table 2). Relative weight gain (RWG) was significantly higher in DCP, MCP, and MHP compared to that in control and TCP (Fig. 1). Fish fed the control showed the lowest P content (0.36%) in whole body, while those fed the MHP the highest (0.46%) after 9-week feeding trial. (Fig. 3). The significant difference in P availability of repetition was not shown from manure analyses every week. Apparent availability of P in the experimental diets varied from 24.2 to 41.9% for TCP and MHP, respectively. The values of P were found to be 93.2, 62.4, 6.1, and 97.8% for MCP, DCP, TCP, and MHP, respectively (Table 3). The available P level in diets (Table 1) was calculated based on determined P digestibility, which ranged from 0.32 (control) to 0.66% (MCP).
Values (means ± SE of triplicates) with different superscript letters in the same row are significantly different (p < 0.05)
ns nonsignificant
1Daily feed intake (%/av. wt/day) = dry feed intake (g/fish)/((initial wt + final wt)/2)/experimental days × 100
2Weight gain (%) = (final weight (g) − initial weight (g)) × 100/initial weight (g)
3Feed conversion ratio = dry feed intake/wet weight gain
4Protein efficiency ratio = wet weight gain (g)/protein intake
5Specific growth rate (%) = (Ln final weight (g) − Ln initial weight (g))/experimental days × 100
6Survival rate (%) = final fish number/initial fish number × 100
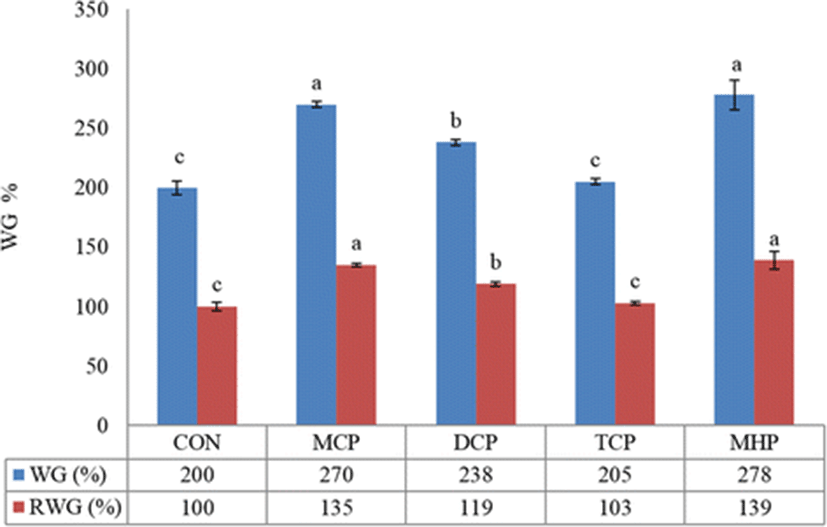
P availability |
|||||
---|---|---|---|---|---|
CON |
MCP |
DCP |
TCP |
MHP |
|
Diet |
26.57 ± 1.07c |
42.27 ± 0.47a |
34.30 ± 0.46b |
22.37 ± 0.74d |
41.97 ± 0.68a |
Phosphorus sources |
93.19 ± 1.96b |
62.42 ± 3.37c |
6.11 ± 2.02d |
97.97 ± 2.06a |
Means ± S.D of three replicates of each group with different superscript letters in the same row are significantly different (p < 0.05)
Hematological parameters and inorganic P in plasma of fish fed the experimental diets are shown in Figs. 2 and 3, respectively. PCV (%) of fish fed TCP (24.2) and control (25.5) showed a significant difference (p < 0.05) from that of fish fed MHP (28.8). Hb (g/dL) of fish ranged from 7.5 (TCP) to 8.8 (MHP), which were significantly different (p < 0.05). Significantly lower P (mg/dL) in plasma was found in fish fed the control (4.3), while the other groups did not show any significant difference (p > 0.05) each other.
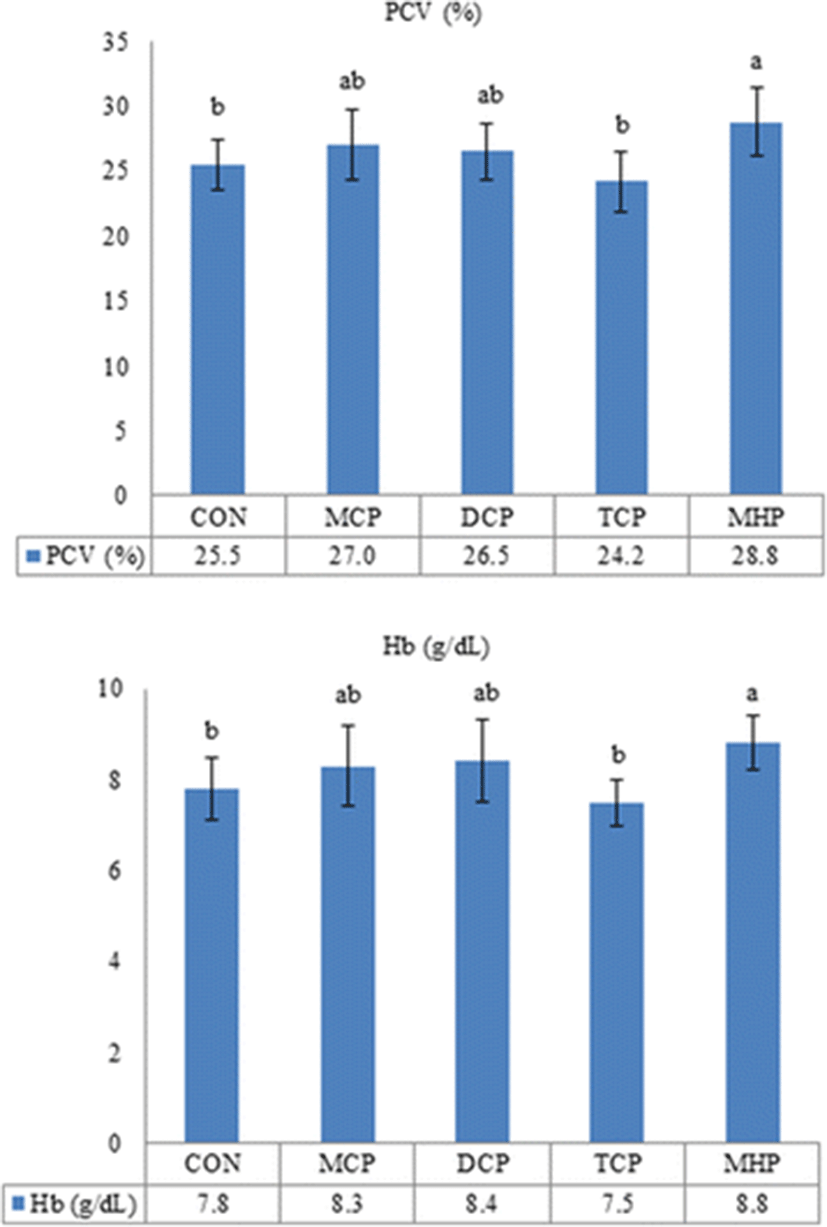
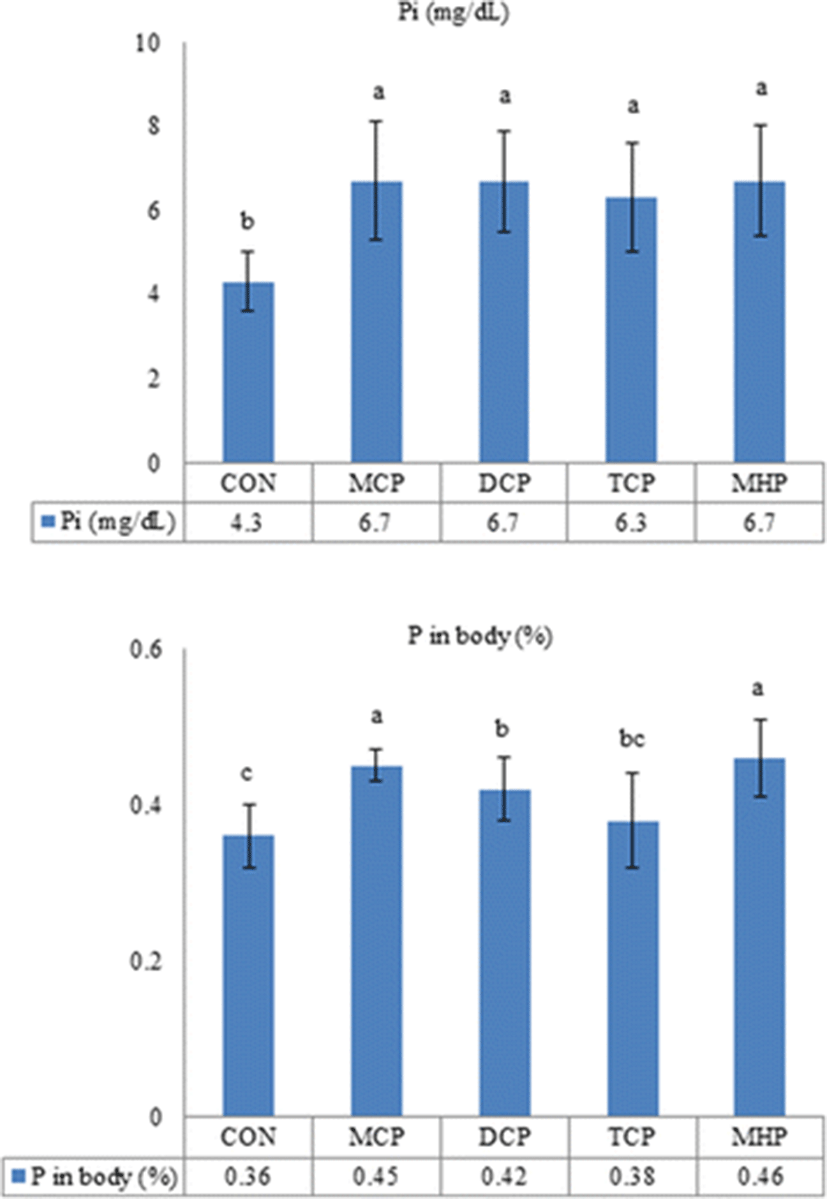
Discussion
As the environmental risks has been increased by releasing animal fecal wastes including fisheries, the P-recovery from swine manure has been introduced in the form of struvite and widely studied (Yoon et al. 2015). Its application has been only limited as a fertilizer but it is much desirable to apply P recovered to new applications after appropriate manufacturing and securing safety. Magnesium hydrogen phosphate (MHP) was newly manufactured from struvite which was to recycle P from swine manure. It is first attempt to investigate the effect of the MHP as dietary P source on growth and feed utilization of carp. Based on the earlier study (Kim et al. 1998), who found that 2% of MCP in diet for carp improved growth and decreased P loads, the same level of various P sources was incorporated into the experimental diets (Table 1). Fish fed the MHP showed the best WG at the end of growth trial, although it was not significantly different (p > 0.05) from that of fish fed the MCP. Same tendencies were found in FCR, PER, and SGR (Table 2). The result suggests that juvenile carp could utilize P from MHP as effectively as MCP. From the results, it was evident that MHP was a good P source competitive with MCP in terms of WG and FCR in juvenile carp. As shown in Fig. 1, RWG of MCP, DCP, TCP, and MHP to control at the level of 2% in each diet were 135, 119, 103, and 139% on WG, respectively. Such relative differences among various P sources might be due to the difference in availability of P, by which the requirement could be met or not. Available P requirement is known to be 0.6~0.7% (Ogino and Takeda 1976; Kim et al. 1998) for carp. The present study confirmed such a requirement to be optimal.
Ogino et al. (1979) extensively studied the P availability of inorganic P sources and various feed ingredients by fish. They reported that the availability of MCP, DCP, and TCP was 94, 46, and 13 by carp, respectively, using egg albumin-based diet. Though somewhat higher values were obtained for DCP and TCP in the present study, the value for MCP corresponds well with their result. On the other hand, MHP had the higher P availability than MCP, suggesting its potential use for carp. Similar results were reported in the previous study conducted using juvenile catfish (Yoon et al. 2014). They found that MHP (90.9%) had P availability comparable to MCP (88.1%) at 2% incorporation level in diet. Sarker et al. (2009) determined P availability of different P sources by yellowtail using albumin-based diet. They incorporated MCP (2.7%), DCP (3.7%), and TCP (3.6%) into the respective diets and obtained P availabilities of 92.4, 59.2, and 48.8% for MCP, DCP, and TCP, respectively. Lovell (1978) reported that the availability was found to be 94 and 65% for reagent grade MCP and DCP, respectively, in channel catfish. On the other hand, Eya and Lovell (1997) determined the net absorption of P from various P sources using all-plant basal diet in channel catfish. They obtained the values of 81.2, 74.8, and 54.8% for MCP, DCP, and TCP, respectively.
To our knowledge, this is the first time P availability of feed grade MCP, DCP, and TCP as well as MHP was determined for juvenile carp. In the present study, P availabilities of both MCP and DCP were found to be high while that of TCP was very low (Table 3). P availability of MCP seems to be comparable to those reported by Ogino et al. (1979), Lovell (1978), Kim et al. (1997), and Sarker et al. (2009). However, it was higher than those reported by Pimentel-Rodrigues and Oliva-Teles (2007) and Eya and Lovell (1997). The availability of DCP was comparable to those obtained in yellowtail (Sarker et al. 2009) and European sea bass (Pimentel-Rodrigues and Oliva-Teles 2007), while it was lower than those obtained in channel catfish (Lovell 1978), rainbow trout (Ogino et al. 1979), and far eastern channel catfish (Yoon et al. 2014). On the other hand, reported P availability of TCP by stomach fish ranged from 42% (Pimentel-Rodrigues and Oliva-Teles 2007) to 64% (Ogino et al. 1979). In contrast, the value from the present study was as low as that obtained by carp, stomachless species (Ogino et al. 1979). Bioavailability of dietary P is influenced by several factors including chemical form, digestibility of diet, particle size, interaction with other nutrients, feed processing, and water chemistry (Lall 1991). It remains to be explained whether such discrepancies in P availability of various P sources, especially TCP, are due to the differences in species and diet composition employed for the digestibility measurement as well as methodological approach in fecal collection (Kim et al. 1996). On the other hand, P availability of MHP was higher than those of MCP obtained from carp and rainbow trout by Ogino et al. (1979) and from yellowtail by Sarker et al. (2009), suggesting that MHP could be a potential P source for fish.
Hematological and serological parameters are useful in monitoring the physiological status of fish and as indicators of the health of the aquatic environment, although they are not routinely used in fish disease diagnosis (El-Sayed et al. 2007). Hematocrit (PCV, %) provides an indirect measurement of the body’s oxygen carrying ability, while Hb (g/dL) a direct measurement of the oxygen carrying capacity of the blood (McClatchey 2002). It was reported that hematological parameters could be influenced by nutritional status (Spannhof et al. 1979), infectious disease (Barham et al. 1980; Iwama et al. 1986), environmental changes (Giles et al. 1984), and stress (Ellsaesser and Clem 1986). The normal ranges of healthy adult Atlantic salmon were reported 44 to 49 and 8.9 to 10.4 for PCV and Hb, respectively (Sandnes et al. 1988). Somewhat lower values for cichlid fish were reported by V´azquez and Guerrero (2007), which were 22.5 to 39.2 for PCV and 5.2 to 8.3 for Hb. Recently, Rahimnejad and Lee (2013) reported 30.7 to 34.3 for PCV and 4.4 to 5.4 for Hb of red sea bream fed various dietary valine levels. Our findings for the parameters are in good agreement with those obtained from tilapia (Hrubec et al. 2000) and striped bass (Hrubec et al. 2001), although there is no available information on the effect of dietary available P on the parameters of fish. In the present study (Fig. 2), PCV and Hb of fish groups fed low available P in diet (control and TCP) were significantly lower than the MHP group (p < 0.05). Differently from the present results, Yoon et al. (2014) observed that dietary low P resulted in PCV significantly lower but did not affect Hb. An increase in available P in diet resulted in an increase in plasma Pi (Vielma and Lall 1998; Bureau and Cho 1999; Avila et al. 2000). A clear evidence was observed by Yoon et al. (2014) that plasma Pi increased in fish fed MCP and MHP with higher available P, resulting in the significant improvement in WG by meeting dietary P need. However, any significant difference in plasma Pi was not found in fish groups fed diets with various P sources (p > 0.05) in the present study. Nevertheless, P in whole body was greatly affected by dietary available P levels (Fig. 3), suggesting successive P intake through diet maintains plasma Pi constant then deposition in skeletal tissues occurs when P requirement is met. More researches remain to be clarify the difference in plasma Pi level observed in previous (Yoon et al. 2014) and present studies.
Conclusions
In conclusion, the potential use of MHP recovered from swine manure was proven sufficiently to replace MCP as an alternative P source with respect to WG and FCR as well as P availability. “Such a re-use of P from swine manure could positively influence on the development of other useful sources from livestock manure.”