Background
Since macroalgae (MA) is one of the most expensive (US$3–4/kg) and highest components (15–30% amount) in formulating commercial abalone feed in Eastern Asia, especially Korea (Jang et al. 2018), development of alternative source that is both cheap and supply-stable for MA needs to continue for effective abalone (Haliotis spp.) culture. MA used as common feed for year-around abalone culture are either Laminaria japonica Areschoug or Undaria pinnatifida Harvey and commonly included into abalone feed depending on their price and availability.
As those MA are, however, exclusively harvested during winter season in wild in Eastern Asia, either dry or salted MA are commonly used for abalone culture for the rest of other season. Abalone farmers prefer feeding abalone on MA over formulated feed due to easy management and convenience of farm in Korea although well-formulated feed produced better growth performance over MA (Bautista-Teruel et al. 2003; Garcial-Esquivel and Felbeck 2009; Jung et al. 2016; Myung et al. 2016; Jang et al. 2018). In addition, an international market price of MA is also expected to increase due to high demand for human consumption and expansion of abalone farm in the future. Therefore, development of an alternative source for MA in abalone feed is highly desirable.
Natural diet of abalones is known to consist of 40–50% carbohydrate, and they have various digestive enzymes capable of hydrolyzing complex carbohydrates (Fleming et al. 1996). Mai et al. (1995a) demonstrated that abalone (H. tuberculata and H. discus hannai) had high potential for utilizing carbohydrate for energy. Abalone utilized carbohydrate better than lipid as an energy source (Britz et al. 1994; Monje and Viana 1998; Thongrod et al. 2003), probably explained by the fact that abalones have high levels of the digestive enzymes of proteases, amylase, alginase, agarase, carrageenase, and laminarinase, but low levels of lipases (Emerson 1967; Gómez-Pinchetti and García-Reina 1993; Britz et al. 1994; Erasmus et al. 1997; Garcia-Esquivel and Felbeck 2006). Therefore, unlike fish utilizing carbohydrate poorly (Wilson 1994; Stone 2003), abalones seem to utilize a variety of plant or crop as energy source in feed well. Reyes and Fermin (2003) reported that the combined terrestrial leaf meal (Moringa oliefera) and freshwater aquatic fern (Azolla pinnata) with animal protein (white fish and shrimp meals) were the promising feed ingredients for the practical diet for the farmed abalone (H. asinina) in the Philippines.
White radish (Raphanus sativus L.) is a widely consumed vegetable crop in Korea as the traditional food, Kimchi, composed of its root and leaves. White radish’ by-product, mainly composed of unsuitable stems and leaves (WRB) for human consumption are dumped and regarded as trash. Leaves of white radish are known to have anti-inflammatory activity in lipopolysaccharide-stimulated macropahages via nuclear factor-kappa B inactivation (Park and Song 2017). Water extracts of white radish seeds have been used in traditional medicine to treat digestive inflammation, diarrhea, and abdomen illness in East Asia (Kim et al. 2015; Choi et al. 2016). Therefore, WRB has high potential as an alternative source for MA in abalone feed. Kim et al. (2016) also reported that Laminaria japonica at 20% in abalone (H. discus) feed was completely substituted with rice bran, which is one of agricultural wastes.
Since tunic of sea squirt (Halocynthia roretzi von Drasche) (TSS) is usually dumped to the sea after de-shelling for human consumption, it is regarded as a pollution source. Annual aquaculture production of sea squirt reached 31,353 tons in 2016 (KOSIS 2017). Kang et al. (1996) demonstrated that the enzymatic hydrolysates of TSS could be used as the protein and carbohydrate sources for aquaculture. Both WRB and TSS seem to have high potential as alternative source for MA in abalone feed.
Therefore, in this study, substitution effect of WRB and TSS for Undaria in feed on growth and carcass composition of abalone (H. discus) was determined.
Methods
Juvenile abalone were purchased from a private hatchery and transferred to an abalone farm (Ocean and Fisheries Research Institute, Jeju Special Self-Governing Province, Jeju, Korea). Before an initiation of the feeding trial, abalones were acclimated to the experimental conditions for 2 weeks and fed with the dry Undaria once a day at the ratio of 1.5–2.5% total biomass. Eight-hundred forty juvenile abalones averaging 5.4 g were randomly distributed into each of the 12, 70-L plastic rectangular containers (120 × 36 cm) (70 per container). Twelve containers were placed into 10-ton concrete flow-through raceway systems (water volume: 2.8 tons) as a flow rate of 48.2 L/min/raceway. The sand-filtered seawater at a temperature ranging from 17.2 to 19.3 °C (mean ± SD: 18.4 ± 0.06 °C) at 17:00 h was supplied throughout the feeding trial. Aeration was supplied into each raceway and the photoperiod followed natural conditions. The experimental diets were fed to abalone once a day (17:00 h) at a satiation level (about 2–3% biomass) with a little leftover. Dead abalones were removed daily and the bottoms of the containers were siphon-cleaned daily. The feeding trial lasted for 16 weeks. At the end of the feeding trial, abalone was harvested and collectively weighed from each container.
Three formulated diets were prepared in triplicate (Table 1). Fish meal, fermented soybean meal and casein were included as the protein source in the formulated diets. Dextrin and squid liver and soybean oils were used as the carbohydrate and lipid sources, respectively, in the formulated diets. A 15% Undaria was included in the control diet. The 15% Undaria was substituted with the same amount of WRB and TSS, referred to as the DRG and TSS diets, respectively. The formulated diets were satisfied for dietary protein and lipid requirements for abalone (Mai et al. 1995a, b; Fleming et al. 1996; Bautista-Teruel et al. 2003). Finally, dry Undaria was prepared to compare effect of the formulated diets on performance of abalone.
aVitamin premix contained the following amount which were diluted in cellulose (g/kg mix): excipient, 317; riboflavin, 23.8; pyridoxine, 4.7; niacin, 95.2; Ca-pantothenate, 33.3; inositol, 476.9; folic acid, 1.5; p-amino benzoic acid, 47.6
bMineral premix contained the following ingredients (g/kg mix): excipient, 45.5; MgSO4, 140.8; NaH2PO4, 92.4; KH2PO4, 246; Ca(H2PO4)2, 139.5; ZnSO4, 22.5; Ca-lactate, 310; AlCl3, 0.15; KI, 0.15; MnSO4, 2; CoCl2, 1
cCarbohydrate was calculated by the difference (100 − % crude protein − % crude lipid − % ash)
Next, a 20% sodium alginate was added to three formulated diets. All the ingredients were mechanically mixed well and water added at a ratio of 1:1. A paste was made from each of the diets by using an electronic mixer and shaped into 0.15-cm thick sheets, which were then cut by hand into 1-cm2 flakes. The flakes were then dipped into an aqueous solution of 5% CaCl2 for 1 min. The flakes were dried at room temperature for 2 days and stored at − 20 °C until use.
Twenty abalones at the start and from each container at the end of the feeding trial were sampled and frozen for chemical analysis and growth measurement. Prior to examination, all samples were slightly thawed, followed by separation of the shell and soft-body tissue. Shell length and shell width were measured in millimeters with a digital caliper (Mitutoyo Corporation, Kawasaki, Japan), and the soft body weight was measured. Specific growth rate (SGR, %/day) was calculated using the formula of Britz (1996): SGR = [(ln(Wf) − In(Wi))/days of feeding] × 100, where In(Wf) = natural log of the final mean weight of abalone and In(Wi) = natural log of the initial mean weight of abalone.
The separated soft body tissue from all abalones from each container was then homogenized and used for proximate analysis. Crude protein content was determined by the Kjeldahl method (Auto Kjeldahl System, Buchi B-324/435/412, Switzerland), crude lipid was determined using an ether-extraction method, moisture was determined by oven drying at 105 °C for 24 h and ash was determined using a muffle furnace at 550 °C for 4 h. All methods were according to standard AOAC (1990) practices.
The three formulated diets and dry Undaria were placed in separate 70-L plastic rectangular containers (120 × 36 cm) without abalone in duplicate. These containers were then placed within a 5-ton concrete flow-through raceway indoor tank at a flow rate of 48.2 L/min and subsampled at 12, 24, and 48 h to evaluate leaching of nutrients in the diets to determine their water stability. Nutrient levels in the diets were assessed using the same procedure described above for abalone carcass. Water stability of nutrients in the diets was expressed as the percentage of final dry content to initial dry content for each nutrient based on Mai et al. (1995a)’s study.
One-way ANOVA and Duncan’s multiple range test (Duncan 1955) were used to determine the significance of the differences among the means of treatments by using SAS version 9.3 program (SAS Institute, Cary, NC, USA). Water stability of the experimental diets was tested by ANOVA with repeated measurement designs (Cody and Smith 1991). Percentage data was arcsine-transformed prior to statistical analysis.
Results
Crude protein, crude lipid, and ash content of the experimental diets were significantly (P < 0.0001) changed over all periods of time (Figs. 1, 2, and 3), and their significant (P < 0.0001) interactions (experimental diets × time) were also observed. After 12 h immersion in seawater, the retention of crude protein content in the TSS diet was significantly (P < 0.05) higher than those in the all other diets and highest and lowest for the TSS diet and Undaria, respectively (Fig. 1). The amount of dry matter content retained in the control and WRB diets was also significantly (P < 0.05) higher than that in the Undaria after 12 h immersion in seawater. The retention of crude lipid in the experimental diets was not significantly (P > 0.05) different at 12 h after seawater immersion, but the retained percent of crude lipid in the all formulated diets was significantly (P < 0.05) higher than that in the Undaria at 24 h (Fig. 2). The proportion of ash content retained in the all formulated diets was significantly (P < 0.05) higher than that in the Undaria after 12 h immersion in seawater and highest and lowest for the TSS diet and Undaria, respectively (Fig. 3).
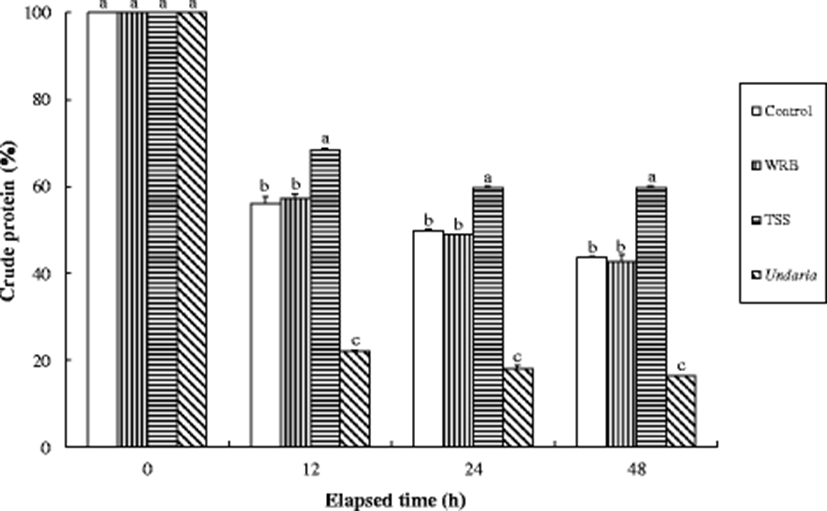
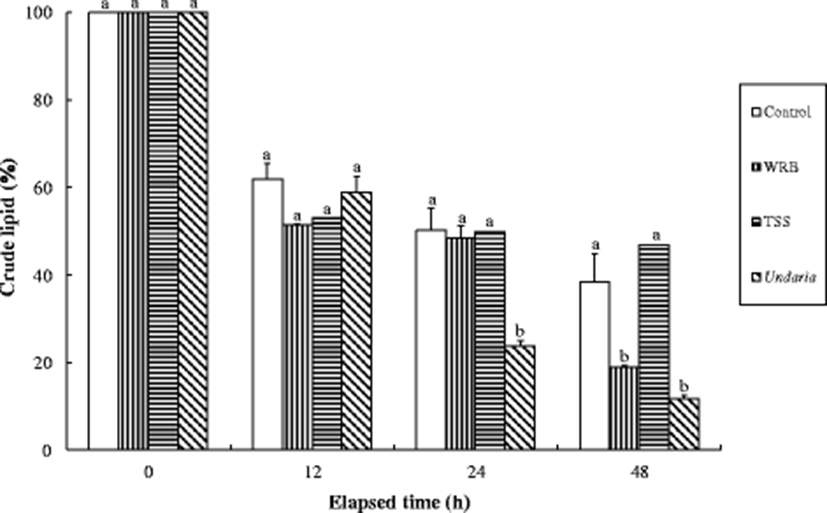
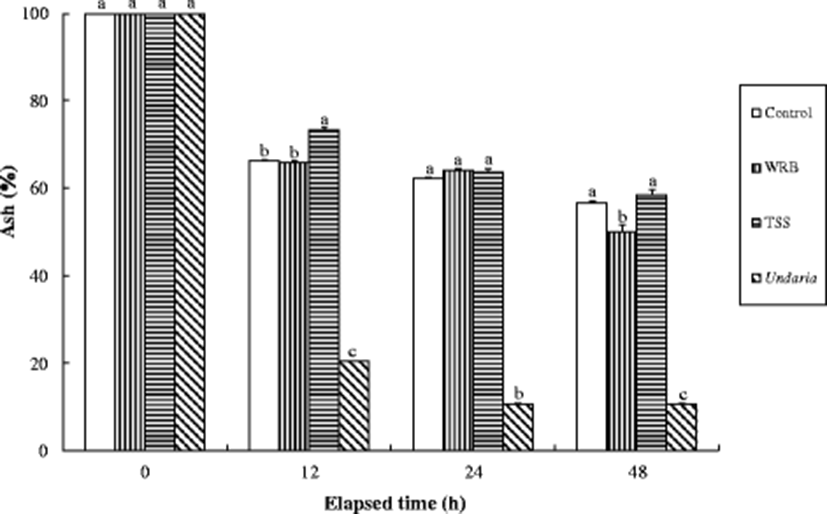
Survival of abalone ranged from 91.4 to 96.2% was not affected by the experimental diets (Table 2). However, weight gain and specific growth rate (SGR) of abalone fed the TSS diet was significantly (P < 0.05) greater than all other experimental diets. Weight gain and SGR of abalone fed the control diet was also significantly (P < 0.05) greater than those of abalone fed the Undaria, but not significantly (P > 0.05) different from those of abalone fed the WRB diet.
Values (means of triplicate ± SE) in the same column sharing the same superscript letter are not significantly different (P > 0.05)
aSpecific growth rate (SGR, %/day) = [(Ln(Wf) − Ln(Wi))/days of feeding] × 100, where Ln(Wf) = natural log of the final mean weight of abalone and Ln(Wi) = natural log of the initial mean weight of abalone
Shell length of abalone fed the TSS diet was significantly (P < 0.05) higher than all other experimental diets (Table 3). Shell width and height was not significantly (P > 0.05) affected by the experimental diets. Soft body weight of abalone fed the TSS diet was significantly (P < 0.05) higher than that of abalone fed the WRB diet and Undaria, but not significantly (P > 0.05) different from that of abalone fed the control diet.
Moisture content of the soft body of abalone fed the control diet was significantly (P < 0.05) higher than that of abalone fed the WRB diet and Undaria, but not significantly (P > 0.05) different from that of abalone fed the TSS diet (Table 4). Crude protein content of the soft body of abalone fed the WRB and TSS diets was significantly (P < 0.05) higher than that of abalone fed the control diet and Undaria. Crude protein content of the soft body of abalone fed the control diet was also significantly (P < 0.05) higher than that of abalone fed the Undaria. Crude lipid content of the soft body of abalone fed the WRB and TSS diets was significantly (P < 0.05) higher than that of abalone fed the Control diet and Undaria. Ash content of the soft body of abalone fed the control and WRB diets and Undaria was significantly (P < 0.05) higher than that of abalone fed the TSS diet. Higher crude protein and lipid content of the soft body of abalone fed the formulated diets compared to those of abalone fed the Undaria was well reflected from dietary nutrient content.
Discussion
Because abalones are slow-eaters, water stability of the diet is critical to determine growth of abalone and severity of water pollution in farms. Higher crude protein and lipid and ash contents retained in the all formulated diets (Control, WRB, and TSS) diets compared to the Undaria at 24 and 48 h after seawater immersion indicated that water stability of the formulated diets was superior to the Undaria. Especially, water stability of the TSS diet seemed to be superior to other diets at 24 and 48 h after seawater immersion in this study. Similarly, water stability of the formulated abalone feed was superior to dry MA (Undaria and Laminaria) at 24, 48, and 72 h after seawater immersion (Lee et al. 2016). Bautista-Teruel et al. (2003) also reported that water stability of the formulated diets was estimated to be 64% at 24 h when animal and plant protein sources was tested for abalone (H. asinina).
SGR of abalone fed the formulated diets ranged from 0.60 to 0.68%/day in this study was comparable to those obtained (0.53–0.60 and 0.61–0.88%/day for the same species of abalone with initial weight of 3.3 and 4.3 g grown at mean temperature of 18.0 and 19.5 °C, respectively) in Lee et al. (2016) and Jang et al. (2018)’s studies and superior to ones obtained (0.45–0.46 and 0.28–0.34%/day for the same species of abalone with initial weight of 0.43 and 1.29 g, respectively, grown at mean temperature of 18.4 °C) in Kim et al. (2016) and Jung et al. (2016)’s studies.
No difference in weight gain and SGR of abalone fed the control and WRB diets indicated that WRB is a promising alternative source for Undaria in abalone feed. This could be explained by the fact of comparable nutritional values [crude protein (CP): 21.6% and crude lipid (CL): 1.3%] in WRB with ones [CP: 28.5% and CL: 0.7%] in TSS (Table 1). Similarly, the agricultural by-product, rice bran, was the good alternative source for L. japonica in abalone feed (Kim et al. 2016). Reyes and Fermin (2003) also showed that locally available year-around terrestrial leaf meal and aquatic fern are the promising feed ingredients for practical diet for farmed abalone (H. asinina) in the Philippines. Since abalone utilize carbohydrate better than lipid as an energy source (Britz et al. 1994; Thongrod et al. 2003) and have high levels of the digestive enzymes (Emerson 1967; Gómez-Pinchetti and García-Reina 1993; Garcia-Esquivel and Felbeck 2006), a variety of terrestrial leaves has worth of evaluating alternative source for MA in abalone feed to lower feed cost. Fleming et al. (1996) also reported that abalone had various digestive enzymes capable of hydrolyzing complex carbohydrates. Feasibility and suitability of WRB as the alternative source for MA in commercial diet on abalone farm, therefore, needs to be proved before practical application (Table 3).
Values (means of triplicate ± SE) in the same column sharing the same superscript letter are not significantly different (P > 0.05)
Improved weight gain and SGR of abalone fed the TSS diet compared to the control and WRB diets in this study indicated that TSS is a superior alternative source to either Undaria or WRB for MA in abalone feed. MA in abalone feed was completely substituted with TSS without retardation of growth of abalone (H. discus) and the maximum weight gain was obtained in abalone fed the diet substituting TSS with 40% MA when TSS was substituted with 0, 20, 40, 60, 80, and 100% MA at 20% in abalone feed (Jang et al. 2018). Feasibility of substitution of TSS with Undaria in commercial abalone feed needs to be tested in a commercial-scale farm. Kang et al. (1996) explained that the enzymatic hydrolysates of TSS could be used as the protein and carbohydrate sources for aquaculture. Extracts of TSS were suitable feed additive for aquaculture use (Lee et al. 1994; Hong et al. 2002).
Difference in both studies [improved weight gain and SGR in abalone fed the TSS diet substituting 100% MA with TSS in this study vs slightly poor weight gain and SGR of abalone fed a diet substituting 100% MA with TSS in Jang et al. (2018)’s study] could be resulted from difference in amino acid balance in the experimental diets in both studies: [assuming no reduction, even slightly increase in crude protein content (essential amino acid) due to no reduction in the main protein source in the TSS diet in the former vs reduction in some essential amino acid, such as arginine, histidine, isoleucine, leucine, lysine, phenylalanine, threonine, and valine due to decreased soybean meal in the diet substituting 100% MA with TSS in the latter] (Table 4). The essential amino acids, such as lysine, methionine, and histidine are the limiting factors to evaluate suitability of alternative protein source for fish meal in abalone feed (Shipton and Britz 2001; Bautista-Teruel et al. 2003; Cho et al. 2008; Cho 2010). Mai et al. (1994) also showed that the essential amino acids (arginine, methionine, threonine, and histidine) were the limiting factors in six species of MA (Ulva lactuca, Chondrus crispus, Palmaria palmata, Alaria esculenta, Laminaria digitata, and L. saccharina) for growth of abalone (H. tuberculata and H. discus hannai).
Values (means of triplicate ± SE) in the same column sharing the same superscript letter are not significantly different (P > 0.05)
Shell length and the soft body weight of abalone were relatively well reflected from growth rate of abalone in this study. Similarly, the biological criteria measured were coincident with growth rate of abalone (Bautista-Teruel et al. 2003; Cho 2010).
Higher crude protein and lipid content of the soft body of abalone fed the formulated diets compared to those of abalone fed the Undaria was well reflected from dietary nutrient content, agreeing with other studies showing that the proximates of the soft body of abalone was directly affected by dietary nutrient content (Uki et al. 1986; Mai et al. 1995a, b; Thongrod et al. 2003; Cho et al. 2008; Garcia-Esquivel and Felbeck 2009; Cho 2010; Kim et al. 2016; Myung et al. 2016).