Background
Temperature is one of the most critically recognized environmental factors to influence almost all the physiological aspects of poikilothermal animals, especially embryonic development and early ontogeny (Güralp et al. 2017). Developmental rates of embryos and larvae depend on incubation/rearing temperatures, and the temperature range at which normal development proceeds is species-specific (Güralp et al. 2017; Wang et al. 1985). Knowledge on the optimal temperature range of egg incubation is essential for successful artificial seedling production in aquaculture, and the application of micromanipulation and/or chromosome-set manipulation to the embryos often requires the specific temperature regime of egg incubation to make these operations effective (Güralp et al. 2017; Nam et al. 2004; Güralp et al. 2016).
The Russian sturgeon (Acipenser gueldenstaedtii) is an emerging candidate in the Korean aquaculture domain, mainly owing to its highly valued caviar (Ossetra caviar) (Kim et al. 2009). Although the Russian sturgeon was first introduced to Korean farm 15 years ago, reproductive control and artificial seedling production of this sturgeon species have been hardly achieved until the mid-2010s. The pioneering work on the artificial fingerling production with the farmed Russian sturgeon broodfish in a Korean farm was first recorded in 2015 (Park 2018). Developmental stages of Russian sturgeon embryos have been outlined previously by hand-sketched illustrations with descriptions on the temperature-dependent development rates (Dettlaff and Vassetzky 1991; Dettlaff et al. 1993). However, with an aquaculture viewpoint on mass production of hatchlings, the embryonic viability incubated at different temperatures has not been extensively studied yet. Furthermore, sturgeons, in particular Russian sturgeon, often represent a considerably wide temporal window of hatching period (i.e., from the first occurrence of advanced hatching to the completion of hatching within a given embryo batch) (Park 2018; Park et al. 2013a). Such a wide window for hatching time occasionally hurdles the synchronization of larval development, consequently making it difficult to decide the best timing to provide first exogenous feed (Gisbert and Williot 1997). Transition to exogenous feeding is a crucial step for successful larval nursery, since this critical phase is immediately followed by a high mortality phase caused by the transient cannibalism (Gisbert and Williot 1997). For this reason, the incubation of artificially produced embryos at optimal temperature at hatchery would be invaluably important for improving the efficiency and capacity of the managing practice for seedling production in this species. We (1) updated the embryonic development of the Russian sturgeon based on the comprehensive photographic data on complete development stages, (2) examined the effects of water temperatures on embryonic viability, and (3) scrutinized the effects of incubation temperature on the temporal window of hatching event.
Methods
Mature Russian sturgeon female broodfish (n = 3; mean body weight [BW] = 22.6 ± 4.5 kg) were given intramuscular injections of the luteinizing hormone-releasing hormone analogue des-Gly10, [D-Ala6] LH-RH ethylamide (LHRHa; Syndel Laboratories Ltd., BC, Canada) at the dose level of 20 μg/kg BW. At 12 h after the primary injection, females were administered again with the LHRHa at the resolving dose of 80 μg/kg BW. On the other hand, male broodfish (n = 4; 18.8 ± 3.9 kg BW) were injected once with LHRHa (80 μg/kg BW) at the same time of the second injection for females (Park 2018). After injection, each fish was maintained in individual incubation tanks (3-ton capacity at 16 ± 1 °C). Females were monitored until a small number of ovulated eggs were released (about 36 h after the second injection), while semen was collected from hormone-injected males with a silicon tube-connected aspirator at 30 h post injection and stored in an extender at 4 °C until use (Park and Chapman 2005). Eggs striped from each female and artificial insemination was carried out by gentle mixing eggs with diluted milt (1/100 dilution of extender-suspended milt with 1-μm filtered, 16 °C freshwater) for 2 min. Fertilized eggs were rinsed with clean freshwater and subjected to the treatment of Fuller’s earth (Sigma-Aldrich, St. Louis, MO, USA) for removing the adhesiveness of the fertilized eggs (Park 2018). Treated eggs were finally rinsed three times with clean freshwater and placed in the incubation chambers adjusted at desired water temperatures.
Two independent spawning trials were carried out in different years (2015 and 2017). Documentation of photographic images for embryogenesis was made at 19 ± 0.5 °C. Embryos (n = 30–40) were sampled with a 1-h interval until 12 h post fertilization (HPF), with a 2-h interval until 40 HPF, with a 4-h interval until 72 HPF, and with a 12-h interval until 120 HPF (first hatch). In addition, several non-periodical samplings of developing embryos were also made when needed. Triplicate samplings were made at each detection point. Sampled embryos were fixed in cold 4% paraformaldehyde. Outer jelly coats of embryos were removed with fine forceps and subjected to microscopic examination in order to determine the developmental stages and also to scrutinize morphological features of the embryos (Park et al. 2013b). Image analysis was made with the NIS-Elements BR image analysis software implemented in the AZ100 microscope (Nikon, Tokyo, Japan).
Effects of incubation temperatures on developmental rates and embryonic viability were examined with egg batches produced in 2015. Fertilized eggs (ca. 800 eggs each) were assigned into one of four incubation chambers at 12, 16, or 20 °C. Water temperatures in incubation chambers were adjusted to be ranged within ± 0.5 °C with thermostat-assisted aquarium 300 W heaters. Dissolved oxygen was adjusted to be 8.0 ± 0.5 ppm. Dead embryos from each incubation chamber were counted and removed every 12 h. Developmental progress and cumulative survival rate at each temperature group were assessed at early blastula, onset of gastrulation, formation of small yolk plug, late neurulation, s-heart formation, and first hatching. Triplicate analyses were performed.
The time spectra of hatching events under different incubation temperatures (12, 16 and 20 °C) were examined with egg batches produced in 2017. Fertilized eggs were incubated at 17–18 °C until 100 HPF (stage for tail reaching s-heart), and embryos were divided into three groups (12, 16, and 20 °C groups). Each temperature group comprised of triplicate batches (n = 500 per replicate batch). After the allocations, the incubation temperature for each group was adjusted to 12, 16, or 20 °C with electric thermostat-assisted cooler (1 kW) or heater (300 W). The temperature decrease/increase rate was 1 °C/h. Development of embryos in each temperature group was further monitored until the first advanced hatch occurred. Upon the first advanced hatch of a few embryos from each temperature group, pre-hatching embryos (n = 240) at tail-beating stage were immediately transferred to each of new incubators (pre-adjusted to 12, 16, and 20 °C) to monitor the temporal pattern of hatching event. Hatched larvae belonging to each temperature group were counted within a 24-h interval. If hatching was no longer recorded in an embryo batch for 48 h, the hatching event of the embryo batch was considered to be complete.
Finally, the optimum temperature regime was validated under a scale-up condition in 2017. Approximately, 70,000 ovulated eggs from two females were inseminated with milt from a single male, and the resultant fertilized eggs were incubated at a selected temperature. Embryonic viability at pre-hatching stage (100 HPF) was determined by examining the survival rate with 200 randomly chosen embryo samples (triplicate samplings). On the other hand, temporal pattern of hatching event at the same incubation temperature were examined from 105 HPF (the first advanced hatching detected) by examining 10,000 tail-beating, pre-hatching embryos. Triplicate examinations were carried out.
Results and discussion
A complete set of photographic images for embryonic development from the fertilization to just before hatching is provided in Additional file 1: Figure S1. Just fertilized embryos (0 HPF) showed an average diameter 3.43 ± 0.11 mm for animal-vegetal axis. Developmental patterns observed in this study were, in overall, accordant with previous descriptions (Dettlaff et al. 1993; Park et al. 2013b), although the onsets and transitions of a particular stage did not exactly match the time points reported in previous works, probably mainly due to the difference in incubation temperatures. Under the present incubation conditions, uneven holoblastic cleavages comprising of irregular blastomeres continued until 10 HPH (Dettlaff et al. 1993). Embryos reached early blastula stage characterized by a primordial cleavage cavity (11 HPF) and the onset of gastrula stage with a typical “dorsal-lip” appearance (18 HPF) (Chebanov and Galich 2011; Bolker 1993). After the process of epiboly cover (22–30 HPF), a slit-like neural groove was formed in the blastopore as a typical sign for the onset of neurulation (34 HPF) (Colombo et al. 2007; Shook and Keller 2008). During neurulation, a pair of rudimentary excretory system appeared as parallel to the central neural groove (Wrobel 2003; Krayushkina et al. 2012), brain rudiment folded, and dorsal-tail region progressively thickened. The neural tube was almost closed at 44 HPF (Lowery and Sive 2004; Vijayraghavan and Davidson 2017). Thereafter, morphogenesis of embryonic body proceeded, with particular highlights on differentiations of heterocercal tail and craniofacial structure. Morphological differentiation of the tail region was characterized by the initial formation of neural groove in blastopore (38 HPF), widened and risen shape (42 HPF), flattened structure with closed neural tube (44 HPF), transformation from flattened shape to rod shape (48 to 56 HPF), straightened shaping with the development of fin fold rudiments and the separation from the yolk sac (64 to 72 HPF), pronounced budding of fin fold (84 HPF), bended caudal end with cloaca rudiment (96 to 102 HPF), and widened and round-shaped fin fold with a typical cloaca rudiment (108 HPF) (Fig. 1). On the other hand, the differentiation pattern of head and craniofacial region could be characterized by the initial formation of neural plate (36 HPF), formation of rudimentary brain part (38 HPF), distinguishable partition of rudimentary brain (42 HPF), thickened and primarily structured head (44 HPF), from round-shaped to angled head with the development of eyes and olfactory sacs (52 to 64 HPF) (Zeiske et al. 2003), and highly differentiated structure with brain cavities and auditory vesicle (68 HPF and afterward) (Fig. 2).
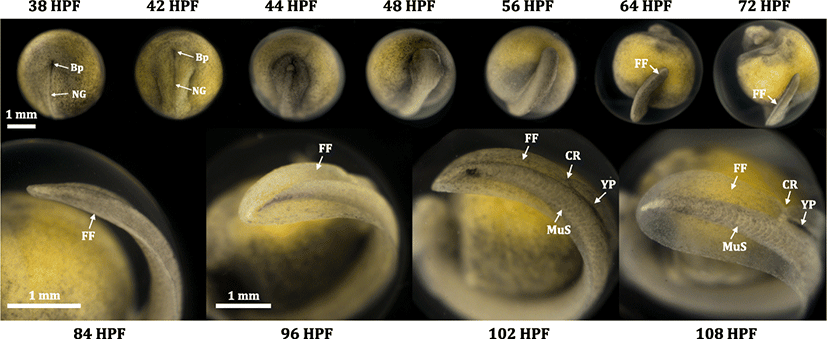
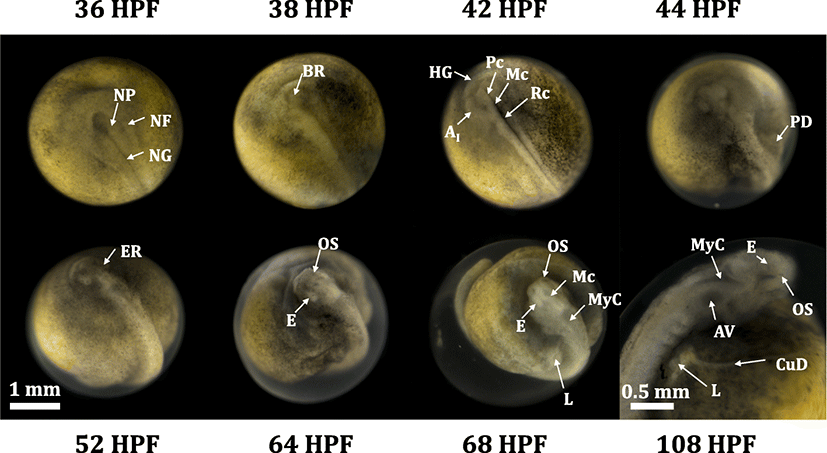
Upon hatching (first advance hatching at 105–109 DPH), prolarvae were 10.6 mm in average total length and the length of yolk (head to tail) was accounted for about 35% of the total length. In overall, newly hatched Russian prolarvae represented common morphological features typically known in prolarvae of Acipenser species (Dettlaff and Vassetzky 1991; Dettlaff et al. 1993). From the dorsal view, a pair of loop-shaped anterior part of pronephros was distinct and fin fold was developed along the dorsal side of the body. Magnified dorsal view also could allow the identification of faintly pigmented eyes (and eye lens), metencephalon and myelencephalon cavities, auditory vesicles (otocysts), rudimentary base for pectoral fin, and branchial grooves (rudimentary structure for external gills) (Dettlaff and Vassetzky 1991; Dettlaff et al. 1993; Park et al. 2013a). From the lateral view, heterocercal tail with well-developed caudal fin fold was characterized. Blood vessel networks appeared mainly in the posterior/ventral part of the yolk sac wall. Pronephric duct was present along the posterior part of the body trunk until the cloaca. Posterior region of the primary digestive track was filled with dense yolk plug (also called pigment plug), which would be eventually evacuated during the transition from endogenous nutrition to exogenous feeding (Park et al. 2013a; Gisbert and Williot, 1997). Magnified viewing of the head region enabled more detailed documentations of brain cavities and eye structure (optic vesicle and eye lens) (Additional file 2: Figure S2).
Developmental times required for reaching selected stages were inversely related with the incubation temperatures (12, 16, and 20 °C) as expected (data not shown), and the pattern was not significantly different from those observed in previous studies (Chebanov and Galich 2011). However, embryonic viability was affected by the incubation temperature, in which embryos incubated at the lowest temperature (12 °C) was significantly lower than were those of two other temperature groups (16 and 20 °C) (P < 0.05). Significant difference between 12 and 16 °C/20 °C groups was first detected at onset of gastrulation, and afterward, the difference became progressively intensified until the first hatch (Fig. 3). This finding is well in agreement with previous recommendations on the temperature ranges (14–18 °C or 16–20 °C) of egg incubation in the Russian sturgeon (Chebanov and Galich 2011). Although reasons for adverse effects at 12 °C incubation on the embryonic viability has yet to be explained, one possible, but untested, explanation is that activities of enzymes and other modulating effectors responsible for normal development (especially for preparing gastrulation and downstream processes) might be insufficiently presented at the low-incubation temperature. Similar phenomenon has been reported in the Siberian sturgeon (Park et al, 2013a); however, adverse effects caused by low temperature might be more severe in the Russian sturgeon than in the Siberian sturgeon.
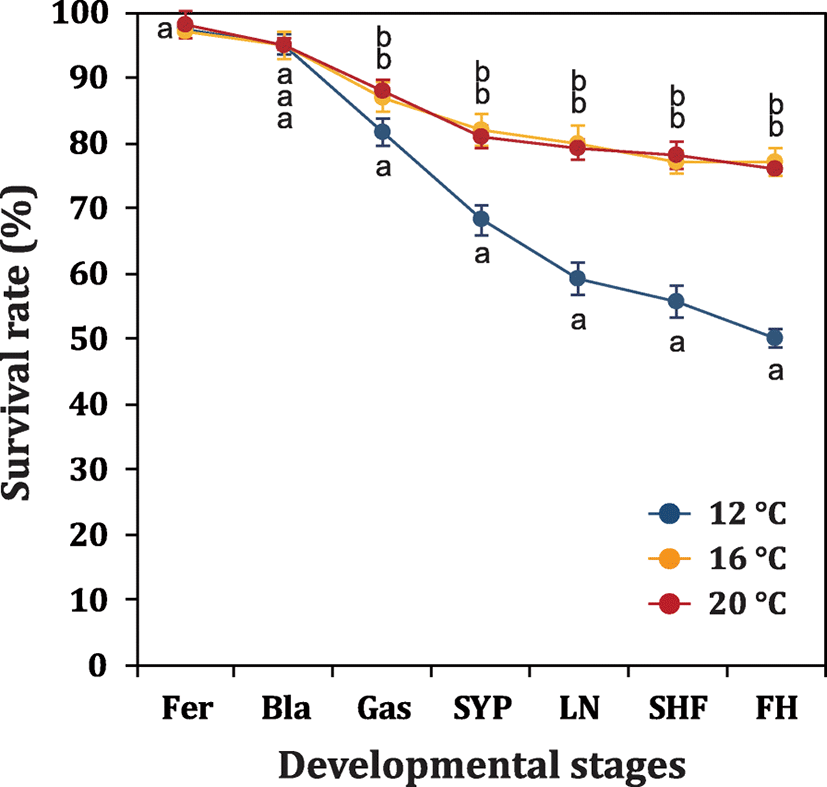
Time spectrum of hatching event (i.e., period from the first hatch to the completion of hatching) was also related to the incubation temperature (Fig. 4). When incubated at 12 °C, the hatching event continued even 10 days after the first occurrence of hatching. Although the highest portion of embryos hatched at day-H5, no apparent peak was evident at 12 °C. Moreover, a considerable portion of embryos (over 40% for each replicate examination) is persistent to be unhatched with retaining their viability until the end of examination (day-H12) in this study. The overall percent hatching success at Day-H12 (no. of hatched larvae/no. embryos initially incubated at Day-H0 × 100) was only 53.7 ± 4.5%. On the other hand, embryos incubated at 16 °C showed relatively narrow pattern of the temporal spectrum of hatching event as compared to 12 °C incubated embryos did. Even though there was still a certain portion of embryos showing delayed hatching (approximately 13% embryos hatched at day-H7 and after), the percentage of such embryos were significantly lower than that observed at 12 °C. Over 60% embryos hatched within a period from day-H3 to day-H5, and hatching event was finished at day-H10 at 16 °C (overall hatching success = 90.0 ± 2.6%). Further increase of incubation temperature to 20 °C resulted in the apparently shortened time for finishing the hatching event. At 20 °C, majority of embryos (up to 80%) could be successful for hatch-out within 3 days (from day-H2 to day-H4), and approximately 9% of embryos hatched the following day (day-H5). However, a few embryos exhibited delayed hatching at day-H6 and day-H7. Consequently, the percent hatching success at 20 °C was 96.7 ± 2.1%. Overall, our findings are well congruent with previous observations made on Siberian sturgeon embryos treated with similar thermal regimes (Park et al. 2013a). However, when compared to the Siberian sturgeon, the effects of low temperature on the lagged hatching events were more pronounced in the Russian sturgeon embryos. The time windows of hatching event at all the three temperatures tested (particularly at the lowest temperature) were apparently wider in the Russian sturgeon than in the Siberian sturgeon (Park et al. 2013a), suggesting temperature dependency on hatching events might be varied among Acipenser species. More magnified effects of temperature on hatchability and timing spectrum of hatching in Russian sturgeon embryos might be, at least in part, in relation with the fact that outer jelly coat of Russian sturgeon embryo would be stickier and tough compared to that of Siberian sturgeon embryos (personal observations). Although the comparative information on the physicochemical characteristics of the outer jelly coat between the two sturgeon species has remained to be further explored, Russian sturgeon eggs has been reported to require larger amounts and longer treatments of anti-adhesive reagent during the preparation of fertilized eggs than has Siberian sturgeon eggs (Park 2018). Lagged hatching and lowered hatchability at low temperatures might also be potentially associated with either incomplete activity or insufficient amount of hatching enzyme. Hatching enzyme is a protease with a choriolytic activity which can degrade the membrane of egg to let the larvae be free. Hatching enzymes have been isolated and characterized from various teleosts (Kawaguchi et al. 2010; Nagasawa et al. 2016) and also from the Bester sturgeon (hybrid of Acipenser ruthenus and Huso huso) (Nagasawa et al. 2015). However, in contrast to the relative richness of information on in vitro activity of hatching enzyme at different temperature ranges (Shi et al. 2006; Pype et al. 2015), the in vivo expression levels and/or enzyme activity under different water temperature conditions have been little studied. Thereby, expression profiling of hatching enzyme from embryos incubated at different water temperatures could be valuable for future study.
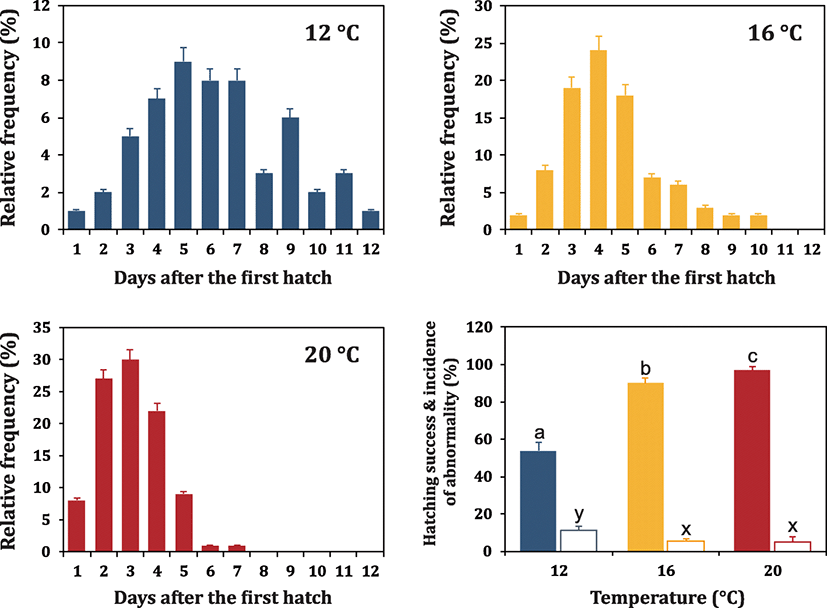
Incidence of abnormality for hatched larvae was also influenced by the incubation temperature. Higher incidence of abnormal larvae was observed at 12 °C incubated groups (11.3 ± 2.3%) compared to groups incubated at 16 °C (5.3 ± 1.5%) and 20 °C (5.0 ± 2.6%) (P < 0.05). Most of abnormal larvae were characterized by bent shape and incapability of normal swimming (photograph not shown), and such abnormal larvae were more frequently observed from the embryos showing delayed hatching especially at 12 °C. Result from this study (i.e., increased incidence of malformed hatchling at 12 °C) is dissimilar from the previous findings from the Siberian sturgeon to have reported no significant difference in abnormality among temperature groups (Park et al. 2013a). Possibly, the difference between these two species might also be related with much more prolonged period of lagged hatching in the Russian sturgeon at 12 °C as compared to the relatively narrower window of hatching time in the Siberian sturgeon at the same temperature condition (Park et al. 2013a).
Patterns for embryonic development and temporal pattern of hatching event at 20 °C were confirmed in a large scale. As a result, the percent embryonic viability at 100 HPF was 76.3 ± 5.6% based on triplicate examinations. Almost all embryos survived until tail-beating stage could hatch-out (hatchability of tail-beating embryos = 95.5 ± 2.1%), and the temporal pattern for hatching event was clearly similar with that observed in a small scale above. In average, 27, 33, 24, and 7% embryos hatched at day-H2, day-H3, day-H4, and day-H5, respectively. Additionally, a small portion of embryos hatched from day-H6 to day-H7, and eventually a few embryos (3–6% depending on replicate examinations) remained unhatched. In overall view, the optimal temperature for the incubation of the Russian sturgeon embryos could be 20 °C regarding the hatchability and/or normality of larvae. More importantly, synchronized temporal window of hatching events at 20 °C could offer lots of advantageous merits for hatchery practices. Under hatchery scale, narrowing the window of hatching period would facilitate the preparation of synchronized larval batch, which would eventually be helpful to decide accurately the initial timing for the supply of the artificial (or the live) feeds to yolk plug-evacuated larvae. Transition from yolk sac nutrition to exogenous feeding in sturgeon larvae has been reported to be accompanied with histochemical, enzymatic, and structural changes of the digestive system (Gisbert et al. 1999; Ostos-Carrido et al. 2009; Babaei et al. 2011). During the large-scale production in hatchery, bacterial and fungal infection from the dead embryos are often unavoidable; thereby, the completion of hatching events in a short period at 20 °C would be desirable for reducing the risk associated with the spread of microbial infections.
Conclusions
A complete set of photographic images for the embryonic development in the Russian sturgeon (Acipenser gueldenstaedtii) was updated, which could be a useful basis not only for developmental studies but also for practical hatchery management of this sturgeon species. Also, our data on effects of water temperature on embryonic viability and hatching times indicate that the egg incubation at 20 °C are recommended in this sturgeon species with regard to the synchronization of hatching events, which is beneficial for the nursery practice of prolarvae in hatchery.