Background
With the advent of health awareness and health-conscious buyers, consumption of seaweeds and related goods has progressively increased in the market. Seaweeds are nutrient-rich food source mainly composed of proteins, amino acids, non-protein nitrogen, lipids, dietary fiber, vitamins, and minerals, as well as various bioactive compounds which are significant for biomedical, pharmaceutical, and health products. Bioactive compounds derived from seaweeds have been studied for anti-inflammatory, antiviral, anti-thrombic, and anticoagulant activities, anticancer and antitumor properties, anti-obesity, anti-diabetic, anti-hypertensive, and anti-hyperlipidemic capabilities (Bedoux et al. 2014; Smit 2004). However, the high demand in seaweed production has resulted to a substantial amount of by-products, which usually end up being dumped back to the ocean, during and after processing (Shi et al. 2019). One example of the commercially cultivated species is Undaria pinnatifida or sea mustards. From the family of brown seaweeds, and locally known as “Miyeok” in South Korea or “Wakame” in Japan, sea mustard is also considered a food commodity in China (Kim and Choi 1994). In seaweed processing industries, sea mustard by-products, such as sporophylls, are found to have essentially the same components to that of sea mustard, even after fermentation, and are examined as potential alternative nutrient source for broilers as animal feedstock (Shi et al. 2019).
Reactive oxygen species (ROS), which include superoxide (O2−) and hydroxyl radicals (OH), and hydrogen peroxides (H2O2) are considered double-edged swords. While playing a crucial role in several biological functions such as intracellular messaging, cellular differentiation, growth arrestment, apoptosis, immunity, and defense against microorganisms, generated ROS may also exceed their antioxidant capacity, disrupt the redox balance, and, therefore, cause oxidative stress which, in turn, leads to cellular dysfunction. Oxidative stress takes a major part in the progression of some ailments like atherosclerosis, hypertension, aging, Alzheimer’s disease, kidney malfunction, and even cancer (Roberts and Sindhu 2009), and accumulated evidence also supported the relationship of increased oxidative stress with diabetes and its complications (Henriksen et al. 2011). Antioxidant supplements have been reported to significantly reduce ROS generation and reinforce immune system defense, consequently preventing and delaying cell damage. Compared to synthetic counterparts, the public prefer natural antioxidants with minimum side effects due to growing health safety concerns (Nishibori et al. 2012). As a result, exploration of prospective antioxidants from plant and marine sources has become a trend, in the hopes of treating degenerative diseases.
Over the decades, fucoidan, a bioactive polysaccharide known for its antioxidant and other anti-proliferative activities, has been isolated from various brown seaweed species Ecklonia cava, Ascophylum nodusum, Laminaria japonica, and U. pinnatifida (Mak et al. 2013; Wang et al. 2009; Hu et al. 2010; Wijesinghe and Jeon 2012). In this study, we investigated fucoidan content from sporophylls of sea mustard from two different locations, Tongyeong and Gijang, in South Korea in order to promote utilization of by-products from Miyeok cultivation and processing, as potential bioactive compounds source, to develop health-related natural products. The isolated crude fucoidan extracts were tested for presence of fucose, protein residue, amino sugars, sulfate (sulfated glycans), and uronic acid and evaluated for antioxidant activities.
Materials and methods
Cultured sea mustards (U. pinnatifida) were collected from the coastal areas of Hansan Island in Tongyeong (TF) and Gijang in Busan (GF), respectively. Sea mustard sporophylls were washed, drained of excess water, and air-dried, protected from direct sunlight. The dried sporophylls were then pulverized using a grinder and kept in zip-locked bags at − 20 °C until further analysis.
Chemical and radical reagents including 1,1-diphenyl-2-picrylhydrazyl (DPPH), nicotinamide adenine dinucleotide (NADH), nitro blue tetrazolium (NBT), phenazine methosulfate (PMS), ethylenediaminetetraacetic acid (EDTA), 1,9-dimethylmethylene blue (DMMB), 3-methyl-2-benzothiazolinone hydrazine hydrochloride (MBTH), and hydrogen peroxide (H2O2) as well as fucoidan from Fucus vesiculosus, D-galactose, bovine serum albumin (BSA), L-fucose, chondroitin-6-sulfate, N-acetyl-D-glucosamine, and D-glucuronic acid standards were all purchased from Sigma-Aldrich (St. Louis, MO, USA). Ascorbic acid and other reagents used were HPLC or analytical grade.
Crude fucoidan was obtained using hot water extraction method by dispersing the defatted sea mustard sporophyll powder in distilled water (1:30 w/w ratio) with constant stirring for 3 h in a water bath at 80 °C. The solution was filtered and the filtrate was neutralized to pH 7.0 before adding anhydrous ethanol at a final concentration of 20% (v/v) ethanol-filtrate mixture to separate alginic acid. The mixture was then centrifuged at 5376×g for 30 min. The collected precipitate was dissolved in distilled water, dialyzed in deionized water for 48 h (MWCO 3600 Da), and lyophilized. The freeze-dried crude fucoidan was further purified by fractionation via anion-exchange chromatography method described by Mak et al. (2013) with minor modifications. Five grams of crude fucoidan was dissolved in 20 mL Tris-HCl buffer (0.05 M, pH 7.4) and passed through a DEAE-Sephadex A-25 column (2.6 × 15 cm), which is equilibrated with Tris-HCl buffer and connected to a fraction collector (Bio-Rad 2110, CA, USA). The first fraction was eluted with Tris-HCl buffer, followed by a step-wise elution with 0–2.0 M NaCl solution at a flowrate of 2 ml/min while eluates, were automatically collected at 4 ml per tube. An aliquot from each eluted sample was checked for presence of sugars using phenol-sulfuric acid method by Dubois et al. (1956) to determine the changes in each elution. Elution was done until no more sugars were present. Carbohydrate-positive fractions were then pooled together, dialyzed in deionized water for 48 h (MWCO 10.000 kDa), freeze-dried, and stored in air-tight containers for further use in succeeding antioxidant activity assays. The crude fucoidan extracts were evaluated for total sugar content, protein residue, fucose, sulfate, amino sugars, and uronic acids using the following methods, respectively: phenol-sulfuric assay with D-galactose as standard (Dubois et al. 1956); Lowry method with BSA as standard; fucose determination with cysteine-sulfuric acid reaction; metachromasia assay with DMMB with chondroitin-6-sulfate as standard (Barbosa et al. 2003); MBTH assay with N-acetyl-D-glucosamine as standard (Tsuji et al. 1969); and carbazole assay with D-glucuronic acid as standard (Cesaretti et al. 2003).
DPPH radical scavenging activity (RSA) of purified fucoidan extracts were analyzed according to slightly modified method by Shimada et al. (1992). Different concentrations (0.01–1 mg/mL) of sample and standard solutions were prepared in 40% methanol, and 4 mL aliquots were mixed with 1 ml of 0.1 mM methanolic DPPH. The mixtures were shaken vigorously and allowed to stand in the dark at room temperature for 35 min. Absorbance was measured at 517 nm. Ascorbic acid served as standard while 40% methanol was used as blank. Fucoidan standard (Sigma-Aldrich, St. Louis, MO, USA) was used as control for comparison. All determinations were performed in triplicate and DPPH RSA was computed using the following equation: DPPH radical scavenging activity (%) = 1 − (A/A0) × 100, where A and A0 were the relative absorbances of DPPH solution with and without standard or sample added, respectively. Lower absorbance of reaction mixture indicated higher radical scavenging activity.
Superoxide RSA assay was performed based on method described by Nishikimi et al. (1972) with some adjustments. Superoxide radicals were generated in the PMS-NADH system of 3 mL Tris-HCl buffer (16 mM, pH 8.0) containing 338 μM NADH, 72 μM NBT, 30 μM PMS, and varying concentrations (0.01–1 mg/mL) of sample or standard solutions. The mixture was incubated at room temperature for 5 min and absorbance was read at 560 nm against Tris-HCl buffer as blank using microplate reader (Spectramax M2 Molecular Devices, Sunnyvale, CA, USA). Analysis was done in triplicates, and ascorbic acid was used as standard and fucoidan (Sigma-Aldrich, St. Louis, MO, USA) was used as control for comparison. Superoxide RSA was calculated using the following formula: Superoxide radical scavenging activity (%) = 1 − (A/A0) × 100, where A and A0 were the relative absorbances of radical solution with and without standard or sample added, respectively. Reaction mixture with lesser absorbance exhibited greater superoxide anion scavenging activity.
Hydroxyl RSA of extracted fucoidan from sea mustard was measured using a modified method by Smironoff and Cumbes (1989). An aliquot (1 mL) of sample and standard solutions with different concentrations (0.01–1 mg/mL) was mixed with 1 mL of sodium phosphate buffer (0.15 M, pH 7.4) containing 10 mM FeSO4, 10 mM EDTA, and 2 mM sodium salicylate and 1 ml of 3% H2O2. The mixtures were incubated at 37 °C for 30 min, and absorbances were measured at 510 nm. Analysis was done in triplicates and ascorbic acid was used as standard, and fucoidan (Sigma-Aldrich, St. Louis, MO, USA) was used as control for comparison. A sample blank was prepared by substituting sample solution with distilled water and replacing H2O2 with sodium phosphate buffer (0.15 M, pH 7.4). Hydroxyl RSA was estimated using the equation: Hydroxyl radical scavenging activity (%) = 1 − (A/A0) × 100, where A and A0 were the relative absorbances of sample and sample blank reaction mixtures, respectively.
Results and discussion
Brown seaweeds are rich in sulfated polysaccharides which are potential functional or nutraceutical ingredients. Seaweed-derived polysaccharides, including fucoidan, have been used in food, pharmaceutical, cosmetics, and other industries (Wijesinghe and Jeon 2012). The extracted fucoidan from sporophylls of sea mustard, U. pinnatifida, from Tongyeong (TF) and Gijang (GF) were 12.1% and 13.6%, respectively. These results were slightly different from the study by Jeon et al. (2012), wherein they assessed 29 alga species and reported yields of 6.2%, 9.2%, 8.8%, and 14.2% crude polysaccharide ethanolic extracts, for sporophyll of U. pinnatifida, U. pinnatifida, Sargassum fulvellum, and Sargassum thunbergii, accordingly, with higher yields (34.8%, 30.8%, and 30.6%, respectively) for Dictyota dichotoma, Capsosiphon fulvescens, and Enteromorpha compressa.
Fucoidan content from seaweeds could vary from species to species, extraction method, geographical location, and seasonal variation (Rani et al. 2017). Aside from species variety, the extraction method used by Jeon et al. (2012) was not specific for fucoidan which could result to other compounds, such as alginic acid, to be included and affect the yield. In another study, Lee YK, Lim, Lee YH, and Park (2006) investigated three cultivars of U. pinnatifida in Korea with a lower yield (1.0–3.8%) of fucoidan extracts while higher but comparable data (13.71–26.34%) was observed for the same species in New Zealand by Mak et al. (2013). Although similar species was used in these studies, the different fucoidan extraction yields may have resulted from different extraction methods utilized and geographical location of the samples. Lee et al. (2006) used dilute acid extraction method which could have affected the extraction rate due to fucoidan’s reduced solubility at lower pH values while Mak et al. (2013) employed calcium chloride extraction.
Bioactive sulfated polysaccharides from brown seaweeds increasingly gained attention in scientific fields, particularly in pharmacology and biochemistry. Functional polysaccharides like fucans and alginic acid derivatives from brown seaweeds have demonstrated a variety of biological properties such as anticoagulant, anti-inflammatory, antiviral, antitumor, and antioxidant activities (Wijesinghe and Jeon 2012). Additionally, anti-apoptotic, anti-rheumatic, and anti-mutagenic properties of seaweeds were revealed to be mediated by their antioxidant activities (Choi et al. 2006). The crude fucoidan extracts were evaluated for total sugar, fucose, protein residue, amino sugars, sulfated glycans, and uronic acid content, as shown in Table 1.
Values are presented as mean ± SD (n = 3)
Total sugar, fucose, protein residue, amino sugars, sulfate, and uronic acid content of fucoidan extract from Tongyeong was composed of 13.27% total sugar, 1.15% protein, 285.57 μg/mg fucose, 708.16 μg/mg sulfated glycans, 253.94 μg/mg amino sugar (hexosamines), and 402.13 μg/mg uronic acids. Meanwhile, components of GF were slightly higher (13.39% total sugar, 1.20% protein, 305.33 μg/mg fucose, 839.83 μg/mg sulfated glycans, 315.95 μg/mg amino sugar (hexosamines), and 470.41 μg/mg uronic acids) than TF (13.27% total sugar, 1.15% protein, 285.57 μg/mg fucose, 708.16 μg/mg sulfated glycans, 253.94 μg/mg amino sugar (hexosamines), and 402.13 μg/mg uronic acids). These data showed the heterogeneous nature of the composition of crude fucoidan extracted from both locations which were relatively comparable to the data in brown seaweed species reported by Mak et al. (2013), for U. pinnatifida, and Liu et al. (2016), for Sargassum pallidum. Additionally, the results confirmed presence of sulfated glycans with fucose residue in the crude extracts. Structural characteristics of fucoidan could be dependent on extraction technique, seaweed species, harvest season, geographical location, and algal maturity (Mak et al. 2013), which could explain the difference in data obtained from TF and GF samples. Presence of protein denoted the inherent nature of fucoidan to retain salts and protein which could be a part of the fucoidan structure or just a mere contaminant. Hayakawa and Nagamine (2009) suggested that pure fucoidan should have 0.1% or less of protein content; therefore, the fucoidan extracts in this study were in their crude forms. Nonetheless, these components influence the bioactivity of the fucoidan extracts from this study (Skriptsova et al. 2012).
DPPH is a stable free radical, typically used to evaluate antioxidant capacity of bioactive compounds in different samples. Antioxidants with hydrogen-donating capability reduce DPPH into a stable diamagnetic molecule, as indicated by the decrease in absorbance at 517 nm (Oktay et al. 2003). As shown in Fig. 1, the scavenging activity of sea mustard fucoidan extracts against DPPH radicals increase with increasing concentrations. DPPH RSA of GF was comparatively higher than TF at all concentrations. At 0.01 mg/ml, DPPH RSA of both TF (4.64%) and GF (9.20%) were higher than the control fucoidan (2.78%). However, as concentration of samples increased to 1 mg/ml, DPPH RSA of control fucoidan (47.15%) was higher than the crude fucoidan extracts (12.84% and 21.47%, respectively for TF and GF). Wang et al. (2009) observed similar results (5–25% DPPH RSA at 0.5–4.0 mg/ml) for synthesized fucoidan derivatives (oversulfated, acetylated, and benzoylated) from L. japonica from Qingdao, China. In addition, relatively comparable DPPH scavenging activities (4–35% RSA at 0.2–1.4 mg/ml) of desulfatedfucoidan fractions from U. pinnatifida, also from Qingdao, China, were reported by Hu et al. (2010). Meanwhile, fucoidan fractions from U. pinnatifida from New Zealand (Mak et al. 2013) exhibited higher, almost double, DPPH RSA than the observations in this study. This may be due to the variation in molecular weight, degree of sulfation, and monosaccharide composition influencing fucoidan’s biological activities (Skriptsova et al. 2012). The fucoidan extracts from this study have molecular weights roughly 10.000 kDa, which is lower compared to the fucoidan fractions from U. pinnatifida from New Zealand (molecular weights ranging from 22 to 171 kDa), which may explain the higher DPPH RSA compared to TF and GF samples. EC50, an indication of the effective concentration of the antioxidant to scavenge 50% of the radicals, of the crude fucoidan extracts were also computed. TF (5.60 mg/ml) was found to have higher EC50 than GF (3.82 mg/ml), which implied that GF was more effective than TF against DPPH radicals.
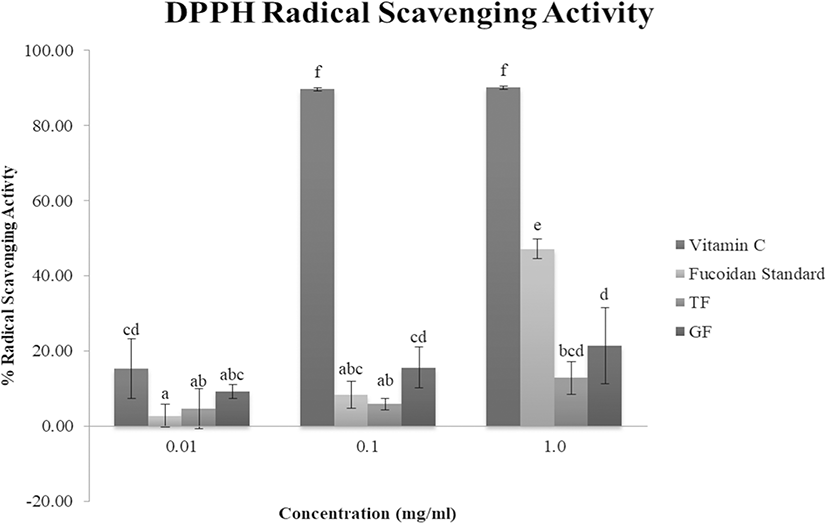
Superoxide anions are considered predecessors of singlet oxygen, hydrogen peroxide, and hydroxyl radicals, thus, indirectly triggering lipid peroxidation, as well as magnifying oxidative cellular damage in lipids, proteins, and DNAs and further intensifying ailments such as arthritis and Alzheimer’s disease (Wang et al. 2009). The scavenging ability of sea mustard fucoidan samples on superoxide radicals were examined in a concentration dependent manner (Fig. 2), and the same trend was observed as with DPPH RSA; superoxide RSA of TF and GF was significantly higher as concentration increased. GF exhibited a notable increase in superoxide RSA from 0.1 mg/ml (29.3%) to 1 mg/ml (39.2%) with EC50 value of 1.63 mg/ml. Meanwhile, TF demonstrated superoxide RSA of 22.6% at 0.1 mg/ml and 26.8% at 1 mg/ml with EC50 of 3.22 mg/ml. These results are slightly lower but almost similar to the data reported by Hu et al. (2010), which range from 10 to 45% RSA at 0.6–1.6 mg/ml of U. pinnatifida fucoidan extract from China. TF and GF were detected to have greater inhibitory effect on superoxide than DPPH radicals, as displayed by their EC50 values. This could be due to superoxide anions being relatively weaker oxidants compared to DPPH and other radicals (Wang et al. 2009). Based on the resulting EC50, GF exhibited greater scavenging ability against superoxide radicals than TF, which may be credited to the higher sulfated glycan contents in GF (839.83 μg/mg) compared to TF (708.16 μg/mg) as electron-donating constituents (like sulfate groups) increase RSA (Wang et al. 2009).
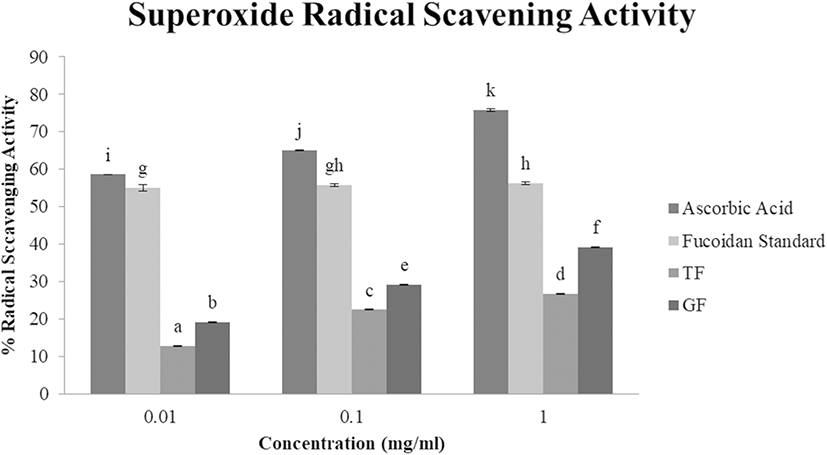
Hydroxyl radicals, OH, are the most active and potent among reactive oxygen species which cause severe damage to neighboring biomolecules like carbohydrates, proteins, lipids, and nucleic acids and initiate aging, cancer, and other degenerative diseases (Chung et al. 1997). Hence, inhibiting or scavenging hydroxyl radicals is vital for antioxidant defense. TF and GF hydroxyl radical scavenging activity were also evaluated in a concentration-dependent manner (Fig. 3). As the concentration increased, TF and GF samples showed greater hydroxyl RSA (TF: 8.4%, 13.4%, and 25.6%, and GF: 10.4%, 14.9%, and 16.6%, respectively, at 0.01 mg/ml, 0.1 mg/ml, and 1 mg/ml). These results were more or less the same to the hydroxyl RSA of crude fucoidan extracted from L. japonica (5–20% RSA at 0.5–2.10 mg/ml) from Qingdao, China (Wang et al. 2009), and moderately lower than that of U. pinnatifida (12–35% RSA at 0.6–1.2 mg/ml), also from China (Hu et al. 2010). Previous studies reported two types of antioxidant mechanism: inhibiting hydroxyl radical generation by formation of metals complexes and scavenging the hydroxyl radicals generated which is related to iron chelating ability (Shon et al. 2003). The observed hydroxyl radical RSA of TF and GF were lower than the control fucoidan (13.7%, 24.8%, and 40.2%, accordingly, at 0.01 mg/ml, 0.1 mg/ml, and 1 mg/ml). Moreover, TF (EC50: 2.53 mg/ml) was found to be more effective against hydroxyl radicals than GF (EC50: 8.40 mg/ml) which could be due to the protein impurities in the extracts. GF (1.20%) was found to have slightly higher protein residue than TF (1.15%) which could have interfered with their hydroxyl RSA.
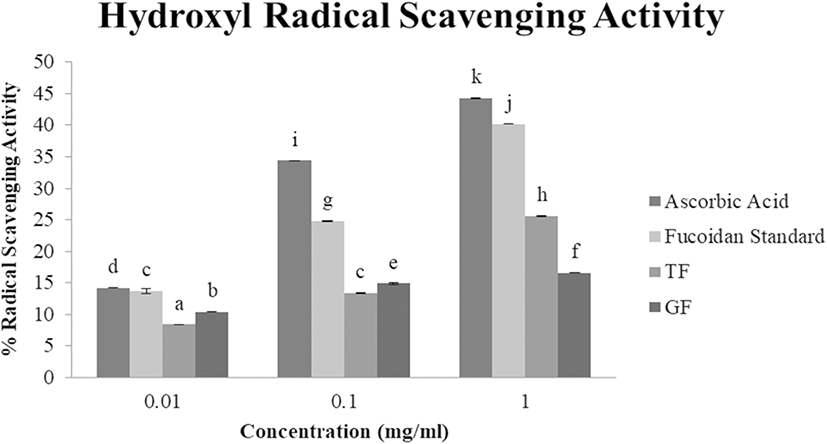
Conclusions
Fucoidan extracted from sea mustard sporophylls from Tongyeong (TF) and Gijang City (GF) both demonstrated lower scavenging activity on DPPH, superoxide, and hydroxyl radicals when compared with ascorbic acid as positive control and pure fucoidan standard as sample control. This could be caused by the impurities present in the crude fucoidan extracted in this study which was supported by the presence of protein residue greater than 0.1%. GF had greater total sugar, fucose, amino sugar, sulfated glycans, and uronic acids content than TF which resulted to GF exhibiting higher RSA against DPPH and superoxide radical. On the other hand, TF displayed higher RSA than GF against hydroxyl radical only which could be caused by greater interfering protein impurities in GF. Further characterization (structure elucidation) and derivatization (acetylation, sulfation, etc.) is recommended to identify the mechanism of antioxidant action in each sample which may improve corresponding bioactivities of TF and GF. Although the crude fucoidan extracts in this study, TF and GF, exhibited lower antioxidant activities compared to ascorbic acid and pure fucoidan, the results showed a prospect of utilizing/exploiting sea mustard sporophylls, which are by-products in seaweed processing,