Introduction
The human population is increasing rapidly and exhausting the Earth’s arable land. Demand for aquatic products is increasing (Cressey 2009). However, advances in fishing technology do not provide more catches but exhaust or exceed the production of renewable fishery resources. Approximately half of all fish stocks have been deemed “fully exploited” or “overexploited” (Cressey 2009). In addition, human activities have drastically reduced the abundance and distribution of marine fish and invertebrate populations through pollution and habitat destruction (Schiermeier, 2002; Pauly and Watson 2003; Islam and Tanaka 2004). Because the world’s fisheries are now known to be in crisis, restocking, and stock enhancement programs have been proposed as a potential method to increase or recover the biomass of depleted fishery stocks (Bell et al. 2008). Stock enhancement has been practiced for over a century, with more than 100 species released to date worldwide (Liao 1997).
Although stock enhancement is considered to be an improved effort toward increasing fishery resources, few cases have been well evaluated or have been substantially evidenced (Kitada 2018). Confirming that changes in fish resources are affected by stock enhancement or other natural factors is a challenge (Blankenship and Leber 1995; Kitada 2018). However, an increasing number of studies have demonstrated that unconscious aquaculture practices and inappropriate artificial release affect the health of natural populations through destruction of habitats, invasion of species, pathogens, and interspecies or intraspecies hybridization. Examples include case studies of the Adriatic sturgeon, Acipenser naccarii (Boscari and Congiu 2014); Korean starry flounder, Platichthys stellatus (An et al. 2014); and black sea bream, Acanthopagrus schlegelli, in Japan (Blanco Gonzalez and Umino 2009). Disruption of genetic structure is a severe problem resulting in reduced population fitness, ecological imbalance, and natural resource shortages (Stokstad 2007; Araki and Schmid 2010).
Taiwan’s government has promoted a massive stock enhancement program in the coastal waters of Taiwan every year, claiming it could enhance the fishery resources. Numerous artificial breeding fry were released, and only a small proportion of fish have been labeled to evaluate their survival rate. In addition, conventional markers (biological, physical, chemical) cannot estimate the reproduction rate of released fish. Because the genetic diversity and genetic structures of natural wild populations must be understood in fishery resource management, molecular markers are useful tools to assist conventional methods applied in stock enhancement programs (Le Vay et al. 2007).
Japan and several countries in Europe and the USA, for example, have official agencies for the management of stock enhancement. They are able not only to verify the reliability of external markers but also to evaluate the genetic composition of offspring and they are reproductively through mark-recapture studies (Bell et al. 2008). Taiwan began to promote the artificial release of seedlings in the 1980s. The government has allocated a considerable amount of money every year but has overlooked genetic factors (Liao et al. 2003). This neglect of population genetics and biodiversity has meant that no well-designed stock enhancement programs were conducted. Official fishery organizations (the Taiwan Fisheries Sustainable Development Association; https://www.tfsda.org.tw/main.php) in Taiwan produce no fish fry directly. The fish fry for stock enhancement are provided by one or several private hatcheries. The source and genetic information of fishes are unknown. Moreover, genetic information for hatchery stocks is also unclear and unstable. Although no genetic information is available for stocks, official organizations perform drug residue analysis for hatchery fry. This provides an opportunity for studying genetic diversity of fish fry and tracing the origins of fish without hatchery information.
As human activities affect wild populations significantly, investigating the genetic structure of wild populations and assessing effectiveness before and after stock enhancement are of critical concern. Therefore, we attempted to use microsatellite markers to discriminate between cultured and wild mangrove red snapper (Lutjanus argentimaculatus) and to examine the possible sources of hatchery-reared fry. This technology was used in the monitoring and evaluating of stock enhancement for the mangrove red snapper in Taiwan to evaluate the effectiveness of this process.
Materials and methods
A total of 787 mangrove red snapper specimens, including 685 hatchery individuals from four batches allocated for release (populations H1, H2, H3, and H4) and 102 individuals from the wild (Kaohsiung and Pingtung; population W), were obtained during 2015 and 2016 (Table 1). Sampling locations with the abbreviated population name and the sample size of each population appear in Table 1. Small pieces of muscle tissues (about 3-5 mm) were prepared from fresh (use of 2% alcohol for anesthesia) or frozen samples, transported to the laboratory for molecular study, and preserved in 95% ethanol. The standard proteinase K/phenol method was modified from an animal DNA extraction protocol. Moreover, 0.8% agarose gel electrophoresis was performed to assess DNA template quality.
N number of samples, Na allele number, Ne allelic richness, Ho observed heterozygosity, He expected heterozygosity, FIS fixation index
A total of seven microsatellite markers (LA01-LA07) were tested (Zhang et al. 2006). Every 4 markers were selected into the same multiplex PCR reaction validated using 10 wild individuals. Loci (LA01, LA03, and LA07) not always got amplicons by using Tm 56-60 °C, and Loci (LA02, LA04, LA05, and LA06) that amplified successfully and stable were combined into further multiplexes. The universal dye-labeled primers used were T3: PET-5′AATTAACCCTCACTAAAGGG 3′, M13 Reverse: NED-5′GGATAACAATTTCACACAGG 3′ Hill: 6FAM-5′ TGACCGGCAGCAAAATTG 3′ and Neomycin rev: VIC-5′ AGGTGAGATGACAGGAGATC 3′. Each forward primer had one of the above universal primer sequences added to its 5′ end (Table 2). PIG-tails were added to the 5′end of all the reverse primers. PIG-tailing leads to an addition of a non-templated adenosine nucleotide to the 3′ end on nearly 100% of PCR products which reduces stutter caused by random addition of dATP (Brownstein et al. 1996). Multiplex PCRs were performed in 5 μl reactions with 50 ng template DNA, 1 × Multiplex PCR Master Mix (Qiagen), 0.2 μM of each reverse primer, 0.05 μM of each unlabeled forward primer (modified with the appropriate universal tail) and 0.2 μM of labeled universal primer for each forward primer labeled with matching universal tail. PCR thermal cycling conditions were as follows: 1 × 95 °C (15 min); 30 × 94 °C (30 s), 56 − 60 °C (90 s), 72 °C (60 s); 8 × 94 °C (30 s), 53 °C (90 s), 72 °C (60 s); 1 × 60 °C (30 min). The fragment analysis of PCR products was performed using an ABI 3130 Genetic Analyzer (Applied Biosystems, USA). The output was analyzed using the GeneMapper software (versions 3.7 and 4.0, Applied Biosystems, USA).
The number of different alleles (Na), the number of effective alleles (Ne), observed genetic diversity (Ho), expected genetic diversity (He), and the fixation index (FIS) were calculated using GENALEX 6.51 (Peakall and Smouse 2012). To elucidate the population genetic structure from multilocus genotypes, the admixture model with correlated allele frequencies was applied using STRUCTURE v2.3.4 (Falush et al. 2003, 2007). Three independent runs were performed for the total data set of K values ranging from 1 to 5. All runs were based on 100,000 iterations of burn-in followed by 500,000 iterations. A graphical representation of the STRUCTURE results was generated using Structure Plot v2.0 (Ramasamy et al. 2014).
Results
Across the four microsatellites, all of the individuals were genotyped successfully. No monomorphic loci were detected among hatchery and wild samples. In total, 28 alleles were detected in all individuals; the marker LA02 exhibited the highest number of alleles in all individuals per locus (15 alleles) and the marker LA05 exhibited the lowest number of alleles in all individuals per locus (2 alleles; Table 1). The marker LA02 in W exhibited the highest number of alleles per locus (13 alleles), whereas the marker LA05 in H1, H2, H3, H4, and W exhibited the lowest number of alleles (2 alleles) (Table 1). Allele richness ranged from 1.928 (marker MSTN2) to 4.656 (marker Pma4) in all individuals per locus (Table 1). The lowest allele richness was 1.018 in H4 (LA04) and the highest allele richness was 7.94 in H2 (marker LA02) (Table 1). Mean estimates of expected heterozygosity for all loci among the five populations were between 0.052 and 0.874 (Table 1). H2 had the highest expected heterozygosity for all the loci (average He = 0.536), whereas H4 had the lowest expected heterozygosity (average He = 0.357; Table 1). The average FIS value among five populations was between 0.255 and 0.571. The highest FIS (0.853) was found in H1 at the LA06 marker, and the lowest FIS (− 0.194) was observed in H3 at marker LA04 (Table 1).
Pairwise comparisons between samples were performed (Table 3). Most comparisons among populations demonstrated high-level genetic differentiation (0.050–0.229) (Table 3). The lowest FST value of 0.016 supports low-level genetic differentiation between H1 and H2.
Structure analysis revealed the best K value (K = 5), which supports five possible clusters among all samples. Most individuals from hatchery populations were grouped into four clusters (red, yellow, green, and blue), and most individuals from the wild population were in the gray cluster (Fig. 1). Only five (0.73%) of the hatchery individuals were grouped into the gray cluster but did not exhibit a high possibility of wild origins (0.53–0.75; data not provided). Structure cluster ratios within each population (red-yellow-green-blue-gray) were H1, 50-3.08-32.31-13.85-0.77; H2, 55.5-3.50-25-16-0; H3, 0-55.42-27.08-17.5-0; H4, 6.09-8.7-77.39-3.48-4.35; and W, 2.94-0-0-0.98-96.08 (Table 4). Four of the samples from wild population (3.9%; 4/102) were revealed to be from a fish farm (Fig. 1).
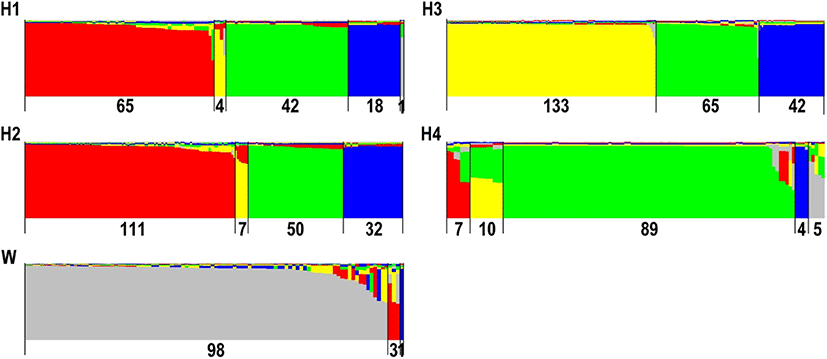

Cluster results were as seen in Fig. 1
N number of samples
Cluster 1, red; Cluster 2, yellow; Cluster 3, green; Cluster 4, blue; and Cluster 5, gray
Discussion
Stock enhancement started in Taiwan with the building and casting of artificial reefs in 1973. However, until 1987, the integrated stock program was only applied for restocking broodstock and fry or seeds. Seven finfish species (5.8 million fry), four mollusks (5 million seeds), and six crustaceans (30 million larvae) were restocked up to 1996 (Liao et al. 2003). Between 2002 and 2018, official fishery organizations (the Taiwan Fisheries Sustainable Development Association) in Taiwan performed stock enhancement of 21 species (20 finfish and 1 crab) with more than 133,593,000 individuals (https://www.tfsda.org.tw). Only a few evaluation cases exist, and the effectiveness of stock enhancement is highly controversial (Hsu et al. 2008). Therefore, the population genetic evaluation method used in this study is not only one of the few evaluation cases but also the first case for the mangrove red snapper in Taiwan.
The mark-and-recapture method is considered to be the most reliable method of assessment. Turbot (Scophthalmus maximus and Psetta maxima), red sea bream (Pagrus major), brown trout (Salmo trutta), and Atlantic salmon (Salmo salar) were used, with the mark-and-recapture method, to examine the contribution rate of stock enhancement (Iglesias et al. 2003; Jonsson et al. 2003; Paulsen and Støttrup 2004; Dahle et al. 2006; Kitada et al. 2009). In Taiwan, taking black seabream (Acanthopagrus schlegelli) as an example, Chang et al. (2011) used a double mass-marking method by spraying fluorescent pigment onto the skin and feeding with oxytetracycline. They marked 105,543 fry (4.6–8.4 cm) for release. The estimated stocking contribution rate was between 2.00% and 9.31% from 2005 to 2008. In this study, four of the field samples (3.9%; 4/102) were revealed to be from a fish farm and most likely from a single batch. It is showing a low contribution rate compared to other research. The contribution rate does not always reflect production enhancement, but it is one of the most essential parameters for stock enhancement assessment. Kitada and Kishino (2006) examined the efficacy of marine stock enhancement for marine fish (red sea bream, Pagrus major and flounder, Paralichthys olivaceus) in Japan. Although the average contribution of hatchery-reared fish was 9.5% for red sea bream and 11.7% for flounder, the production change of wild populations does not appear to be linked to stock enhancement activities. This means hatchery fish might replace wild fish instead of augmenting total production.
The main drawback of the mark-and-recapture method is expensive, time-consuming, and unfeasible for all types of released species such as crustaceans and for early life stages, such as embryo or larvae. Therefore, assessment of stock enhancement with genetic markers was considered a possible solution for red sea bream (Pagrus major), spotted halibut (Verasper variegatus), Pacific herring (Clupea pallasii), Japanese flounder (Paralichthys olivaceus), Japanese bitterling (Tanakia tanago), Chinook salmon (Oncorhynchus tshawytscha), channel catfish (Ictalurus punctatus), black sea bream (Acanthopagrus schlegelii), red drum (Sciaenops ocellatus), and Chinese shrimp (Fenneropenaeus chinensis), for example (Sekino et al. 2005; Ortega-Villaizan Romo et al. 2006; Simmons et al. 2006; Blanco Gonzalez et al. 2008; Eldridge and Killebrew 2008; Hsu et al. 2008; Kubota et al. 2008; Shikano et al. 2008; Kitada et al. 2009; Katalinas et al. 2018; Song et al. 2018). Most studies have evaluated genetic diversity or genetic differences in hatchery and wild populations but have not assessed the contribution rate for stock enhancement. We used the population genetic method not only for examining genetic diversity but also determining the contribution rate of hatchery origin specimens.
In this study, genetic diversity and genetic differences between hatchery and wild populations were evident from the molecular marker. For genetic diversity, He in the wild population (0.536) is higher than in hatchery populations (0.357–0.475) (Table 1), meaning hatchery populations used for stock enhancement would reduce the genetic diversity of wild populations. Some fish such as red sea bream (P. major), brown trout (S. trutta), and black sea bream (A. schlegelii) were observed to have a possible negative effect on wild stock (Blanco Gonzalez et al. 2008; Hansen et al. 2009; Kitada et al. 2009). We observed high-level genetic differentiation (0.050–0.229) between hatchery and wild populations and also among hatchery populations (Table 3). This means that stock enhancement potentially changed the genetic structure of wild stocks. Hansen et al. (2009) analyzed historical and contemporary samples of brown trout (S. trutta) to compare the genetic structure of wild populations before and after stocking with hatchery trout. All populations were observed to be strongly affected by stocking.
Despite the genetic effects of high-level genetic differentiation between hatchery and wild populations, high-level genetic differentiation allows cultured individuals to be discriminated from wild populations after stock enhancement. Assessing the contribution rate of stock enhancement is possible. Structure analysis suggests that four clusters (red, yellow, green, and blue) belonged to hatchery populations (680 individuals; 99.27%) and that one cluster (gray) belonged to a wild population (98 individuals; 96.08%) (Fig. 1). It also suggests high-level genetic differentiation between hatchery and wild populations (Fig. 1). Because four clusters (red, yellow, green, and blue) belonged to hatchery populations, four individuals (4/102; 3.92%) from the wild population might have been hatchery-reared (Fig. 1). Furthermore, we could suppose that these four individuals (three red and one blue) were from H1 and H2 (Fig. 1). Although official organizations implement stock enhancement, they do not produce fish fry directly. The seeds for stock enhancement are provided by one or several private hatcheries. Where the source and genetic information of fish are unknown, our results indicate that genetic information for hatchery stocks was mixed, and a complicated stock source is suggested. H1 and H2 demonstrated a similar composition but not H3 and H4. Structure cluster ratios within each population (red-yellow-green-blue-gray) may facilitate the tracing of origins after stock enhancement (Table 4 and Fig. 1). Official organizations’ drug residue analyses for hatchery fry to be released provide an opportunity to study the genetic composition of the fry and trace the origins of fish lacking hatchery information.
The mangrove red snapper is a prevalent species for stock enhancement (fifth among 21 species in Taiwan), with 6,534,000 individuals released (https://www.tfsda.org.tw/main.php). Without assessment of the contribution of released populations, whether stock enhancement provides benefits is uncertain. The negative effects of stock enhancement are evident in many cases. The main effects include lower survival and growth rates, diminished reproductive fitness, and decreased genetic diversity (Hansen et al. 2009). Few studies have provided direct evidence that wild stock has increased due to hatchery stocking (Kitada 2018). Competition between wild and stocked fish may reduce wild populations and hinder their replacement (Kitada 2018). Lower genetic diversity and high-level genetic differentiation often indicate genetic management risks. These genetic considerations should be carefully weighed during the design of stocking plans, particularly for fish originating from private hatcheries and lacking genetic information in Taiwan.
Conclusions
This study demonstrates that hatchery stocks were admixture with the complicated genetic sources. Before the release, the same batch of fry may originate from various hatcheries. By contrast, the genetic composition of the wild population is relatively simple, and the low contribution rate of hatchery stock (3.92%) indicates that the genetic effect after stock enhancement is weak or nonexistent. Wild mangrove red snappers are genetically different from those originating from farms, and their origins can be traced through molecular markers, even without information on breeding stocks.