Introduction
Yeosu Bay, located in the central part of the southern coast of Korea, is a shallow waterway less than 40 m depth, surrounded by the Dolsan Island and Yeosu Peninsula in the west side, and Namhae Island in the east side. It consists of several bays such as Gamak Bay, Gwangyang Bay, and Jinju Bay which are connected to Seomjin River Estuary. The bays and the river estuary are supplied with water by Seomjin River from the north and rich nutrients from the industrial complexes in the Gwangyang Bay. Among other factors, the warm streamers from Tsushima warm waters and the coastal waters in the southern part of the Yeosu Bay provide suitable environmental conditions for fish migration, fish breeding site and habitat. Therefore, the southern part of Yeosu Bay is an important one of the main fishing grounds in the southern coast of Korea, where many fisheries are operated such as: set nets, gill nets, fish traps, and other types of fishing gears targeting seasonal fishery (Kim et al., 1989; NIFS, 2004). The set net is the most popular fishery in the Yeosu bay, with approximately 30 large set nets in between Dolsan Island, Gumo Islands and Namhae Island (Yeosu City, 2020). It is a passive fishing method that induces fish to enter a set net, traps and then catches them; therefore, it is mainly dependent on migratory fish behavior and the environmental conditions of water masses etc.
There have been several studies on the correlation between water conditions and fluctuation of fish species composition and catches, using the catch data of set nets in some parts of the Yeosu bay. The Yeosu Bay waters is well known for having various oceanic conditions that create fish habitats for spawning and nursing throughout the year (Choo, 2002; Choo & Kim, 1998; Gong, 1971). Temperatures and salinity conditions affect migration, species composition, and catch as catches in the Yeosu bay increase during the period when water temperature and salinity fronts are formed strongly in the coastal region. For example, around the Dolsan Island the best fishing grounds are formed at the boundary between the coastal low salinity water and the southern offshore high salinity water (Kim, 1993; Kim & Rho, 1993, 1994, 1995, 1996; Kim et al., 1988, 1989). On the southern coast of Namhae Island and the Dolsan Island, water temperatures above 17°C (summer and autumn) stimulate the migration of main fish species such as Spanish mackerel (Scomberomorus niphonius) and Hairtail (Trichiurus lepturus), and subsequently increases the catch amount of two species. On the other hand, in spring (below 17°C), there is an increase in sand lances and anchovies species (Cha, 2009; Jeong et al., 2005; Hwang et al., 2006; Shin et al., 2017).
To the best of our knowledge, only a few studies have been conducted on a detailed correlation between water temperatures and the fluctuation of dominant fish species composition and catches in the Yeosu Bay. Therefore, for a better understanding, more studies are required. This study aimed to investigate the correlation between surface water temperatures and the change of fish species composition and catch by a set net (Fig. 1) in the Yeosu Bay.
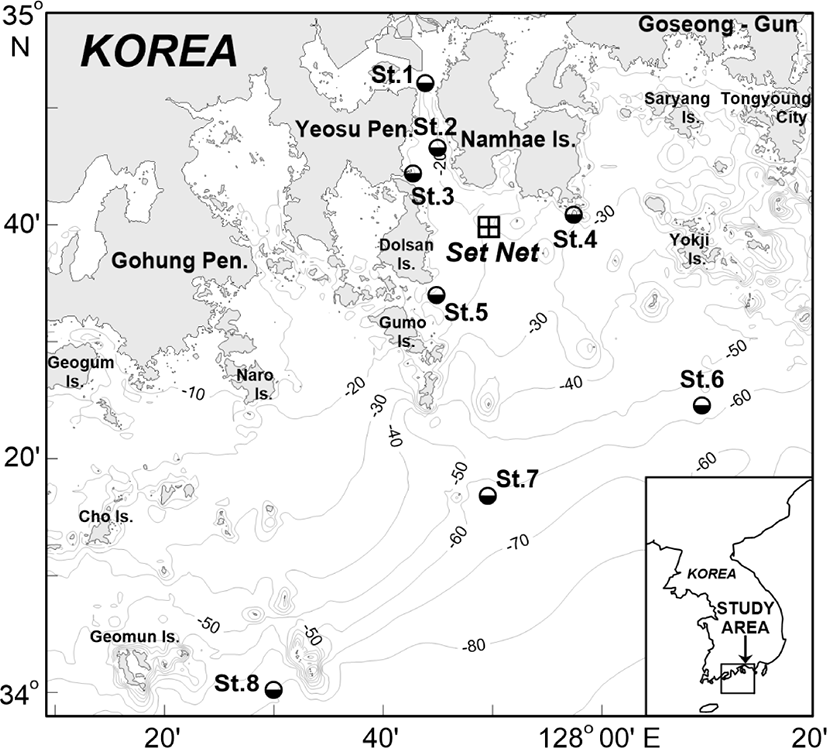
Materials and Methods
Data for fish species composition and the catch of the dominant fish species were obtained from the daily trading records of the set net fishery from April to December in 2016–2018. The fishing grounds are located in the entrance of the Yeosu Bay between Dolsan Island and Namhae Island, approximately 7 km off east of Gye-dong Port in Dolsan Island and are 18m depth (Fig. 1). The fisheries system is constructed over a surface area of approximately 15 hectares. The set net used is 450 m long and 200 m wide, and the length of the leader net leading fish into the fishing gear is 500 m. The set net is composed of a leader net, an entrance, a slope, a bag net and a final trap.
Since the daily trade records of fishing in the set net are recorded mainly for commercial catch, the classification of fish species is targeted only for major commercial fish species. Therefore, the analysis of these data is for the whole day and the dominant species for the major commercial species. Using the daily data, we calculated the monthly mean species composition and catch by dividing the total number by a species with the number of fishing days every month. The sea surface temperature near the fishing grounds was measured at the intervals of 30 minutes to 1 hour at the eight water temperature monitoring stations (Fig. 1). Daily mean water temperature data were used as time series data for analyzing the environmental condition of fishing ground. For maximum accuracy, monitoring stations of St.1 to St.3 in the northwest, St.4 to St.5 in the south and east of the set net, and St.6 to St.8 in the offshore areas were selected. According to Choo & Kim (1998), Gong (1971), and Kim & Rho (1996) fishing productivity around these areas is largely affected by temperatures due to the interaction between the Seomjin River waters and offshore waters flowing toward Dolsan Island from the south of the open sea. Through the statistical analysis of water temperature and catch, the maximum, minimum, average and standard deviation for the period of catch were investigated. The seasonal (April to December) fluctuations of catch and water temperature were analyzed through harmonic analysis (Kang, 2000) using the least-squares method. 3 days low-pass filtering and 30 days high-pass filtering were performed for removing short and long-period component from the daily time series data of water temperature and catch. The 3 days low-pass filtering was performed to reduce fluctuating components in short periods due to external force such as inertial motion, internal wave and small disturbances. Spatial distribution of time series data, spectral density analysis, and coherence (correlation) analysis were used to analyze the correlation between catch of the set net and surface water temperature.
Results
The research period was between April and December, every year from 2016 to 2018. This period included the main fishing seasons which occurs twice in a year (from June to July and October to November). Table 1 presents the results of the monthly catch change by species between 2016 and 2018. The total annual catch was 174.6 tons in 2016, 233.8 tons in 2017, and 179.1 tons in 2018 and three-year average has 195.8 tons. The total catch in 2017 was approximately 25% more than those in 2016 and 2018. During the 3 years, the dominant species was Spanish mackerel, occupying more than 45% of the total catch. Other common fish species were anchovy (Engraulis japonicus), pomfret (Pampus echinogaster) and hairtail accounting for about 10% of the total annual catch. Therefore, the monthly fluctuation of the total catch is largely influenced on the fluctuation trend of the Spanish mackerel.
Fig. 2 represents the monthly catch fluctuation of Spanish mackerel and the others from 2016 to 2018. The fluctuation in catch varies slightly from year to year, but generally indicates an increase in spring (June to July) and fall (October to November). The increase in spring catch is because of the increase in fish species other than Spanish mackerel, and the increase in fall is because of the increase in Spanish mackerel catch. Unlike in August to October 2016 and 2018, the monthly mean catch was 20.5 to 20.6 tons respectively, this was lower than the monthly mean catch for the same period in 2017. The increase in the catch in 2017 was mainly due to the increase in Spanish mackerel species in summer and autumn, however, the catch size of Spanish mackerel during this period was small, about 25 to 35 cm.
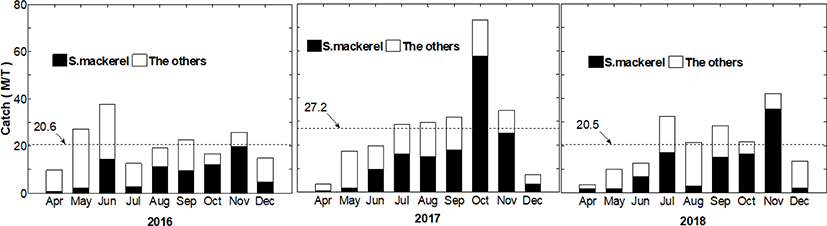
Fig. 3 shows the time series of daily total catches for all species (T. Catch) and daily catches of the dominant species (Spanish mackerel, S. Catch) as well as the time series of the surface water temperature at St.1 to St.8. The T. Catch and S. Catch time series vary depending on the year, but the two-time series show peak 2 times a year, from June to July and from October to November. There appears to be no significant difference in the pattern of fluctuations between the two time series. In particular, the fluctuations in the two time series of T. Catch and S. Catch in 2017, which had high catches, showed the same fluctuation throughout the year except for the period between April and May. Therefore, it can be concluded that the overall catch characteristics of the set net are greatly influenced by whether or not the Spanish mackerel flowed into the fishing grounds when the catch is larger than the previous year.
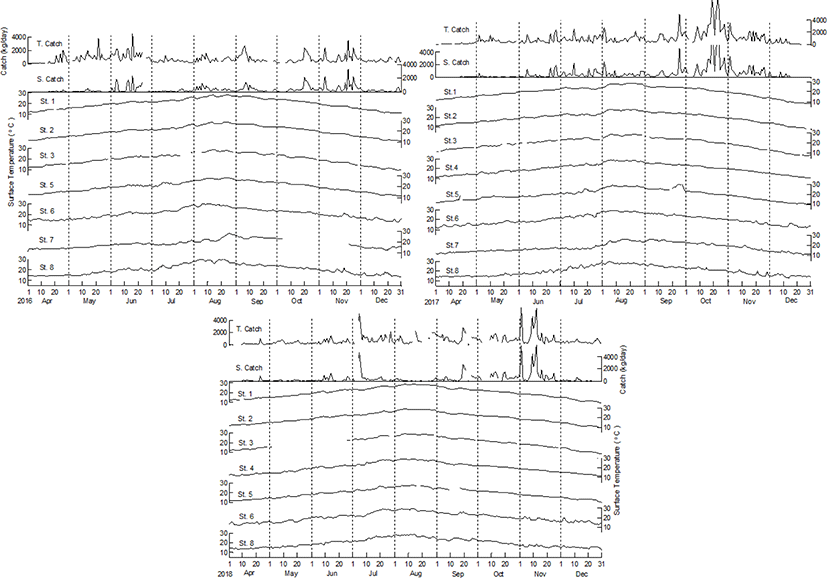
The surface water temperature at St.1 to St.8, unlike the catch, has a distinct seasonal fluctuation, starting to rise around 12°C in early April, reaching the maximum of approximately 28°C in mid-August and then falling to approximately 7°C in late December. Seasonal change due to solar radiation is remarkable at all stations, and especially at St.1 to St.5, these areas, which are estimated to be greatly affected by the shoreline due to shallow depth, have distinct seasonal changes. On the other hand, from the water temperature time series distribution in Fig. 3, it can be seen that the short-term water temperature fluctuations around a week are more pronounced at St.6 to St.8 located in 50 to 60 m depth than at the shallow coastal stations. In addition to fluctuations due to changes in solar radiation, short-term fluctuations in water temperature around 7 to 10 days sometimes occur at St.6 to St.8, located about 50 to 60 m deep in the offshore waters.
Table 2 shows the annual minimum, maximum, mean and standard deviation of the catch and water temperature time series. In the statistical analysis in Table 3, the standard deviation (SD) was the lowest in 2016, ranging from 556 to 406 kg/day, with an annual mean of 695 to 288 kg/day. However, in 2017, the standard deviation was the highest from 990 to 912 kg/day, and the annual mean was 894 to 534 kg/day. This means that the larger the annual catch, the greater the annual fluctuation of the inflow of the population. When the ratio of coastal settled fish population to total catches is large, the yearly catch fluctuations are small and the standard deviation is small. However, if the catch rate of the migratory fish population is large, the annual average is large and the annual standard deviations also increase. In fact, in 2016, the proportion of fish species other than Spanish mackerel in total catches was largest (Table 1).
The water temperature is 3.9°C to 9.1°C in winter(December), 27.4°C to 29.0°C in summer(August), and 16.4°C to 17.4°C on average at the coastal stations (St.1 to St.5). However, in offshore stations (St.6 to St.8), the water temperature ranges from 8.6°C to 11.4°C in winter, 26.2°C to 30.0°C in summer, and 16.1°C to 18.4°C on average. Therefore, the water temperatures at the offshore stations are 2°C to 5°C higher in winter and 1°C lower or higher in summer and on average than the coastal stations. Because the standard deviation is from 5.8°C to 7.3°C in St.1 to St.5 and from 4.5°C to 5.5°C in St.6 to St.8, the annual fluctuation of surface water temperature in coastal area is greater than that in offshore area. The sea area with a small standard deviation of water temperature was stations 7 to 8, which ranged from 4.5°C to 4.9°C, and station 3 had the highest, at 6.8°C to 7.3°C.
Table 3 shows the amplitudes and phases of the annual and semi-annual fluctuations in the total catch for all species (Total. cat), the catch of Spanish mackerel (S. mack. cat), and surface water temperatures at St.1 to St.8. Fig. 4 shows the comparison between fluctuation curves by the harmonic constants of the annual and semi-annual fluctuations of the catches and the surface water temperature at St.5 (costal) and St.8 (offshore). In 2017, the annual fluctuation of the total catch had the largest amplitude and the slowest phase (Table 3). The year with a large catch amplitude was the period when showed the largest total catch for all species and the largest catch of Spanish mackerel. The amplitude of the annual fluctuation of water temperature in 2017 was higher than those of in other years. It is indicated that the amplitude of fluctuations in the catch is high during the period when the intrusion of warm waters is strong. Excluding the total catch in 2016, the annual phase of water temperature is approximately 40° to 50° (30 to 38 days) faster than that of the catch (Table 3). This means that the maximum catch occurs about one month later after the rise of the surface water temperature during the spring-autumn period. The annual fluctuation of water temperature is the largest at St.3 and smallest at St.7 to St.8, the phase is fastest at St.3 and slowest at St.6 to St.8.
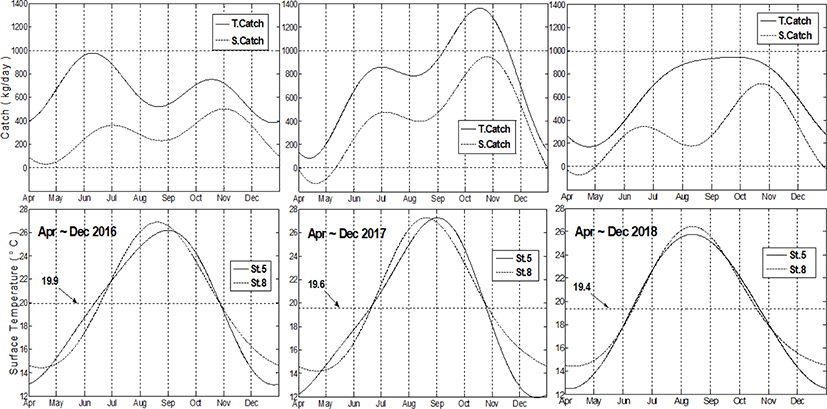
In 2016 and 2017, the total catch (T. Catch) for all species and the catch of Spanish mackerel (S. Catch) gradually increased from May to June when the water temperature in offshore (St.8) is lower than in the coast (St.5), reaching the maximum value at the end of June (Fig. 4). However, from July to August, the offshore water temperature was higher than that of the coast and the catch is generally decreased. Later, from September to October, the offshore water temperature was lower than that of the coast From November to December, the water temperatures in offshore rises higher again than the water temperatures at the coast, and the catches again decrease as in July to August. In 2018, unlike 2016 and 2017, the total catch is generally constant with no decrease in July to August or an increase in September to October. In the spring (May to June) and the autumn (September to October) of 2018, the coastal (St.5) water temperatures were lower than in the previous year, and the highest coastal water temperatures were in early August 20 days earlier than in the previous year.
Table 4 shows the results of the analysis for the predominant fluctuation period of the total catch of all species, the catch of Spanish mackerel and water temperature at station 1 to station 8 during the study period. The predominant period was analyzed from the results of spectral density with 30 days high-pass filtered time series data, and the frequencies of fluctuations with the predominant (1st to 6th) density were converted into fluctuating periods, and the number of occurrences of each period was represented by the frequency (Freq.). Catch fluctuations are predominant in three period ranges: 3 to 4 days, 11 to 15 days and 21 to 28 days. The water temperature is predominant in four fluctuating period ranges: 4 days, 6 to 8 days, 14 to 16 days and 21 to 28 days.
The time series with seasonal components removed from the catch and water temperature time series is shown in Fig. 5. The fluctuations in water temperature and catch (total catch of all species and Spanish mackerel catch) have a time lag, but in the mid- to long-term, the catch increases according to the rising water temperature. In particular, the positive correlation between catch and surface water temperature is remarkable in the water temperature to rise from May to June and drop in July and September 2016, and the water temperature rises from October to November 2016 to 2018. Although there is a difference in amplitude in the long- and short-term fluctuations of water temperature in the figure, the periodic pattern of fluctuations in water temperature and the catch is more consistent as the water temperature monitoring station moves from the coast (St.1 to St.5) to the offshore (St.6 to St.8).
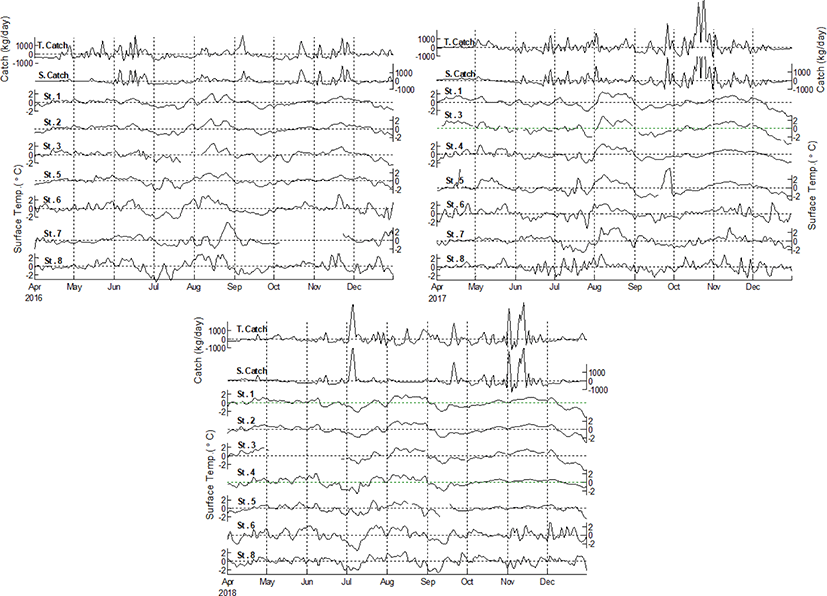
To determine the correlation and phase difference between the two time series of surface water temperature and catch, the coherence (0–1.0) by period (T = 1 / f, f is frequency) of each 30-day high-pass filtered time series and the time lag (τ, τ = θ / 2πf) due to the phase difference(θ) between the time series were analyzed. The fluctuation period obtained by cross-spectral analysis between the catch (total catch of all the species or the catch of Spanish mackerel) and the surface water temperature at each monitoring station is the most predominant at 13.8 day. Table 5 shows the correlation (coherence) and time lag for the predominant 13.8 day period. In the analysis, when the time lag is negative (–), it means that the phase of the water temperature fluctuation is slower than the catch. The St.8 temperature recordings were the most correlated with catch fluctuation, its value was 0.33 (average from 2016 to 2018). Station 7 has a lower correlation (0.15–0.25) than St.8, but it is higher than the rest of the water temperature monitoring stations. The time lag has negative (–) signs in both total catch and Spanish mackerel catch at St.8 only and is less than 1.0 days except in 2017.
Discussion
The set net catches between Dolsan Island and Namhae Island in the Yeosu Bay vary greatly depending on the location of the fishing ground, net sizes, operating conditions and sea conditions (Cha, 2009; Kim, 1993; Kim & Rho, 1993, 1994, 1995, 1996; Kim et al., 1988, 1989, 2013). The dominant fish species in the set net in our study was Spanish mackerel. Spanish mackerel catch starts to increase in May, the highest catch rates are in the period between October and November, then sharply decreases from December. The small-sized Spanish mackerels, whose catches were rapidly increasing from August to October 2017, are believed to be a new stocks that hatch in the East China Sea in spring and enter coastal fishing grounds (Nishikawa et al., 2014). The active recruitment of these new population of Spanish mackerel is also seen in the set nets along the Japanese coast from September to October every year (Inoue et al., 2007). In this way, Spanish mackerel is distributed year-round in the coastal and offshore waters from East China Sea to the South East Sea of Korea, where the Tsushima Current flows. This indicates that the Tsushima Current are very influential on the inflow of Spanish mackerel into the Yeosu Bay’s set net fishing grounds. According to Kim et al. (2013) in the 2004–2011 survey of the catch and species composition of set nets at the southeastern Dolsan Island, Spanish mackerel predominantly accounted for approximately 70% of the total catch from summer to late autumn. Similarly, our study showed that the Spanish mackerel catch rate was high in summer and autumn. Therefore, it is thought that the ecological characteristics of the population of Spanish mackerel should be considered important for the fishing characteristics of the set nets in Yeosu Bay.
As a characteristic of the sea around the fishing grounds, it is known that the area around St.3 east of Dolsan Island, which has a large standard deviation and has a large fluctuation in water temperature. In summer, surface water in the region around St.3 has high temperatures and low salinity while lower layers and the offshore waters have low temperatures and high salinity (Kim et al., 1988). In addition, there is a southward flow that flows along with river water from the north, and a westward flow from the south of Namhae Island towards Dolsan Island, forming a frontal area where coastal and offshore waters come into contact (Kim & Rho, 1994). In the summer, when the mainstream of Tsushima current is strong and the main shaft of warm current approaches the coast, a warm streamer flows counterclockwise from the southern sea of Namhae Island towards the coast of Dolsan Island to form cyclonic circulation in the surface layer around St.3. At this time, it is known that the coastal water fed by river water rich in nutrients, flows down from the north of Dolsan Island into a cyclonic circulating flow, and the middle and bottom waters are mixed by the surface wake (swirl). This is known to provide suitable conditions for biological production (Choo & Kim, 1998). Expectedly, the results of our present study showed that St.3 east of Dolsan Island had a large fluctuation and a high standard deviation of water temperature. The Yeosu Bay, where the set nets were established, is considered to correspond to the frontal area, where two offshore waters and coastal waters come into contact to form a dynamic mixture.
The surface water temperature in coastal areas where tidal current and wake causes vertical mixing has a smaller annual fluctuation amplitude than the other areas. However, among the coastal areas (St.1 to St.5) with strong vertical mixing, the surface water temperature at St.3 has the largest fluctuation amplitude and standard deviation of water temperature. This could be attributed to the stratification of sea water which results from coastal water and offshore waters periodically coming into contact at the region around St.3 (Kim & Rho, 1994). The fact that the surface water temperature phase at St.3 is the fastest of all the temperature monitoring stations also indicates that the region is the most affected by river water.
In general, the offshore water temperature is less affected by seasonal solar radiation than the coast. During winter the offshore has higher water temperature than the coast, while in the summer the temperature is low. Therefore, the seasonal fluctuation of water temperature at the offshore is smaller than at the coast. However, in St.6 to St.8, the offshore surface water temperature is equal to or higher than at the coast due to the seasonal inflow of Tsushima warm water from the south of Geomun Island. When the Tsushima Current in the South Sea of Korea is weak, the water temperature from April to August is generally lower at the offshore (St.6 to St.8) than at the coast (St.1 to St.5), but from September to December, the offshore water temperature is higher than at the coast (Choo, 2002). However, when the Tsushima Current approaches the coast from spring to summer, the offshore water temperature rises above the coastal water temperatures. In the present study, the difference between coastal and offshore water temperatures in June and October 2016 to 2018 can be attributed to the inflow of warm waters from the Tsushima Current. The catch amount tends to rise when the water temperature around the fishing grounds rises and decreases when it decreases. In the future, long-term research is needed to investigate the correlation between the difference in water temperature between the coast and the offshore and the catch using the set net fishing method.
The place where the set net is established is an area where tidal flow prevails and the periodic flow changes between 14 and 28 days due to the tide are predominant. A previous study through infrared images revealed that inflow of warm streamer goes on for several days to a week. The warm streamer plays an important role in transportation and distribution of the fish eggs and larvae spawned and grown in the East Chinese Sea to the South Sea of Korea along the Tsushima Current. In fact, it is often found in surface water temperature infrared photography tracking has shown that the frontal eddy-type warm water flows into the coast every 3 to 4 days from the thermal front formed between the coastal waters and the offshore waters of the South Sea of Korea in spring and autumn (Choo, 2013; Choo & Kim, 1998).
The water temperature at St.1 to St.5 periodically fluctuates when the Tsushima Current becomes strong and the warm water in the form of a frontal wave or cyclonic eddy flows from the offshore into the coast. Qiu et al. (1988) conducted a three-dimensional numerical model experiment of unstable waves formed between the Chinese coastal waters and the Tsushima warm current waters in the East China Sea. In this study, he argued that through a 2 to 7 day baroclinic instability process, the small disturbances on the front side go through the non-linear cascading process and grow into large scale unstable waves toward the coast, and the spatial scale of the unstable eddy (wave) is about 50 to 100 km. It is very difficult to confirm the growth period of an unstable wave appearing in the ocean by simply observing. However, Griffiths & Pearce (1985) reported that it took 10 to 15 days for small perturbations in the Leeuwin Current to grow into a fully developed eddy. In our present study, the periods of catch fluctuation could be attributed to the fluctuations of the sea conditions during eddy, tide, and tidal currents. Our present study also demonstrated that the fluctuation of sea conditions and fish catches are correlated. On the other hand, in the South Sea of Korea coast, where the spectral energy density is high, 4 to 8 day, there are periodic fluctuations suspected to be caused by fluctuations in atmospheric factors such as wind and sea level pressure (Choo & Yoon, 2017), which often occurs in the dominant fluctuation of monsoon prevailing on the coast of South Sea of Korea. Therefore, the short-term fluctuations of water temperature and catch less than 15 days may be as a result of combined effects of the sea conditions and the atmosphere.
Understanding the correlation between ocean water temperatures, physical and environmental parameters, and fishery resources give a clear picture on how ocean conditions fluctuation affects fish catch. Information such as migrating period, migrating place, fish characteristics (length, obesity, gonad status, and main fish species), fishery resource index, catch per unit effort (CPUE), fishery resources, and the correlation between the sea condition and the composition and size of the fish population provides important data for use in the fishery industry to predict catch fluctuations (Japan Society of Fisheries Oceanography, 2014). For the fluctuation of about 14-day period, our coherence study showed a strong correlation between fish catch and surface water temperature in St.7 and St.8 (Table 5), indicating that water temperature is a major factor in catch fluctuations and the temperature fluctuations at St.7 to St.8 located in the offshore have the greatest influence on the set net fishery. In addition, the time delay due to the phase difference between the total catch and the water temperature of St.8 was within –1.0 days (the increase in catch is about 1.0 days ahead of the increase in water temperature), indicating that the two time series are fluctuating almost at the same time in a period of 14 days. However, there was a time lag of 3.0 to 3.5 days during the period of high catches in 2017, and the size of the fisheries population is also considered as a factor affecting the change in the catch. Surface water temperature monitoring station 8, which showed the greatest correlation between fish catch and water temperature fluctuations, is the area through which the Tsushima Current flows in spring and autumn. The temperature fluctuations at St.8 are more affected by fluctuations in sea conditions caused by the Tsushima warm water (Choo & Kim, 1998). Therefore, it can be estimated that the increase in the catch of Spanish mackerel in the set net is due to the coastal inflow of warm waters (surface water temperature rise due to Tsushima warm water) produced by the fluctuations of the Tsushima Current flows in the offshore, which fluctuates every 14 days. These our results give an insight into the prediction of catch fluctuations of the set net in the Yeosu Bay, Korea.