Introduction
Amino acids (AAs) act as regulators of key metabolic pathways and serve as precursors of important biomolecules such as hormones, nucleotides, enzymes, cofactors, antibodies and neurotransmitters (Li et al., 2007). AA deficiencies may delay important physiological processes, highlighting the importance of knowing the specific AA requirements for formulating cost-effective practical diets (D’Mello, 2003).
Threonine (Thr) is an essential AA (EAA) in fish (Feng et al., 2013) and is considered the third limiting AA (after lysine and methionine) in aquafeeds when high levels of plant protein sources are used (Gao et al., 2014; Small & Soares, 1999). Thr is a precursor of non-EAAs (serine and glycine) (Michelato et al., 2016), participates in protein synthesis (Chen et al., 2017) and promotes growth (Zhao et al., 2020) and intestinal health of fish (Dong et al., 2022). It also enhances immune function (Ramos-Pinto et al., 2017) and plays an important role on energy metabolism and nutrient absorption (Kim et al., 2021; Tang et al., 2021). Thr exerts intestinal homeostasis in fish by regulating intestinal morphology, immune response, gut barrier function and gut microorganisms (Dong et al., 2017; Dong et al., 2022).
Many previous studies demonstrated beneficial effects of Thr on growth, immune response, digestion, feed utilization and survival of fish species (Fatma Abidi & Khan, 2008; Michelato et al., 2016). Zhao et al. (2020) observed that dietary Thr increased mRNA expression of IGF-1, myogenic regulatory factors (MRFs) and growth hormone resulting in increased growth and feed efficiency in hybrid catfish (Pelteobagrus vachelli ♀ × Leiocassis longirostris ♂). Thr promoted protein synthesis and upregulated the expression of target of rapamycin (TOR) signaling pathway in Jian carp (Cyprinus carpio var. Jian) (Feng et al., 2013). Similarly, Zhao et al. (2020) demonstrated that dietary Thr regulated the protein kinase B/TOR signaling pathway, thus improving fish muscle protein content. Thr is needed to synthesize mucin in gastrointestinal secretions (Li et al., 2009), enhances thymus growth and activity, and stimulates the immune system (Ahmed, 2007). Gao et al. (2014) observed that dietary Thr affects intestinal serosa thickness and villus height of grass carp (Ctenopharyngodon Idella). Thr deficiency caused growth retardation and reduced feed intake (FI) in Atlantic salmon (Salmo salar) (Bodin et al., 2008) and Pacific white shrimp (Litopenaeus vannamei) (Zhou et al., 2013).
Olive flounder (Paralichthys olivaceous) is the most important commercial marine finfish species in South Korea. Quantitative AA requirements of this fish have only been verified for methionine, arginine and lysine (Alam et al., 2000; Alam et al., 2002; Forster & Ogata, 1998). Further, effects of leucine (Kim & Lee, 2013), isoleucine (Rahimnejad & Lee, 2014) and taurine (Kim et al., 2017) on the growth, biochemical and immune response were demonstrated. To our knowledge, no previous research has quantified the dietary Thr requirement of olive flounder. Therefore, this study was conducted to estimate the dietary Thr requirement of olive flounder based on the growth, survival and feed utilization efficiency.
Materials and Methods
Six experimental diets were formulated to be isonitrogenous (50% crude protein) and isolipidic (10% crude fat) (Table 1). For the basal diet (Thr0.4), a crystalline AA mixture (without Thr) was used as the primary protein source. Six test diets were formulated including graded levels of Thr (Ajinomoto, Shanghai, China) at 0%, 0.4%, 0.8%, 1.2%, 1.6% and 2.0% in the basal diet (designated as, Thr0.4, Thr0.8, Thr1.2, Thr1.8, Thr2.0 and Thr2.3, respectively, based on actual Thr levels). The gradually increased Thr levels were compensated by the decrease in glycine which is a non-EAA. Brown fish meal was added to the semi-purified diets to enhance the diet palatability. The dry ingredients, fish oil and distilled water were thoroughly mixed using a mixer machine (NVM-14, Daeyung, Seoul, Korea) and the wet dough mixture was extruded by a pelletizer (SP-50, Kum Kang Engineering, Cheonan, Korea). Pellets were dried at 25°C for 8 hours and then stored at –20°C until used. The AA composition of the diets is shown in Table 2.
Arginine 6.68, histidine 2.67, isoleucine 4.49, leucine 8.30, lysine 10.86, methionine 2.95, phenylalanine 4.44, tryptophan 0.67, valine 5.41, aspartic acid 10.34, glutamic acid 15.15, serine 3.78, proline 3.26, glycine 7.50, alanine 9.99, tyrosine 3.51.
MgSO4·7H2O, 80.0; NaH2PO4·2H2O, 370.0; KCl, 130.0; ferric citrate, 40.0; ZnSO4·7H2O, 20.0; Ca-lactate, 356.5; CuCl, 0.2; AlCl3. 6H2O, 0.15; Na2Se2O3, 0.01; MnSO4·H2O, 2.0; CoCl2.6H2O, 1.0.
L-ascorbic acid, 121.2; DL-a tocopheryl acetate, 18.8; thiamin hydrochloride, 2.7; riboflavin, 9.1; pyridoxine hydrochloride, 1.8; niacin, 36.4; Ca-D-pantothenate, 12.7; myo-inositol, 181.8; D-biotin, 0.27; folic acid, 0.68; p-aminobenzoic acid, 18.2; menadione, 1.8; retinyl acetate, 0.73; cholecalciferol, 0.003; cyanocobalamin, 0.003.
Experimental protocols were approved by Animal Care and Use Committee of Jeju National University (IACUC, 2019-0031). Juvenile olive flounder were obtained from a hatchery, then kept in rearing tanks and supplied with a commercial diet (Suhyup Feed, Uiryeong, Korea; protein 52%) for ten days to be acclimated to the experimental facilities and conditions. Juvenile fish (bodyweight 23.2 ± 0.4 g) were distributed randomly to 18 circular tanks (215 L each) by stocking 25 fish per tank. Flow-through system was used to supply filtered saltwater to the tanks at a rate of 3 L/min and continuously aerated the water to maintain enough dissolved oxygen levels. Each diet was assigned to triplicate groups of fish and fed twice a day (08.00 and 16.30 h) until satiation. Feeding trial was conducted for 12 weeks and the number of fish and total biomass in each tank were measured every four weeks. The water temperature was maintained at 23.6 ± 3.9°C, dissolved oxygen (9.67 ± 0.07 mg/kg) and the photoperiod was controlled by a 12 h:12 h (light/dark) schedule throughout the feeding trial. Uneaten diets were siphoned out 30 min after each feeding and reweighted after being dried to calculate feed intake. The fish count and biomass of each tank were measured every four weeks. Feeding was stopped for 18 hours prior to the weighing to reduce stress on the fish. Fish were counted and individually weighed in each tank to determine growth performance and survival.
Three fish were randomly selected from each tank and anesthetized with 2-phenoxyethanol (200 mg/L) for sampling. Blood samples were taken and kept to clot at room temperature for 30 min and centrifuged at 5,000×g for 10 min to separate the serum. From another three fish, blood was sampled with heparinized syringes and centrifuged to separate plasma. Plasma and serum samples were stored at –80°C for determination of innate immune and biochemical parameters. Two fish per tank were stored at –20°C for whole-body AA composition analysis.
For non-specific immune parameters, serum lysozyme activity was measured using the method described by Hultmark et al. (1980) with slight modifications. A 200 μL of Micrococcus lysodeikticus suspention (0.75 mg/mL) in sodium phosphate buffer (pH 6.4, 0.1 M), and 20 μL of serum were placed in a 96-well plate, and incubated at 37°C for 60 min. The absorbance was recorded at 570 nm, 0 and 60 min. Hen egg white lysozyme (Sigma-Aldrich, Manngeim, Germany) was used for the standard curve. Values are expressed as μg/mL. Serum myeloperoxidase (MPO) activity was measured using the method described by Quade & Roth (1997). A 20 μL serum was diluted with 80 μL of Hanks balanced salt solution (Sigma-Aldrich, Missouri, USA) in 96-well plates. Then, 35 μL of 20 mM 3,3’,5,5’-tetramethylbenzidine hydrochloride (Sigma-Aldrich, Missouri, USA) and 5 mM H2O2 were added. The reaction was terminated after 2 min by adding 35 μL of 4 M sulfuric acid. MPO activity was expressed as the absorbance at 450 nm. Serum antiprotease activity was measured according to the method described by Magnadóttir et al. (1999). A 20 μL of serum was incubated with 20 μL of 5 mg/mL trypsin solution at 22°C for 10 min. Then, 200 μL of phosphate buffer (0.1 M, pH 7.0) and 250 μL azocasein (2%) (Sigma-Aldrich, Missouri, USA) were added and incubated at 22°C for 1 h. Then, 500 μL trichloroacetic acid (10%) was added to terminate the reaction and kept at room temperature for 30 min. The mixture was centrifuged at 6,000×g for 5 min, and 100 μL of the supernatant was transferred to the 96 well plate. Then 100 μL of 1 N NaOH was added and mixed well. Optical density was read at 430 nm. For positive control, serum was replaced with buffer and for negative control, both serum and trypsin were replaced by buffer. The trypsin inhibition percentage was calculated as, (Absorbance of positive control – Absorbance of the sample) × 100) / (Absorbance of the positive control).
Analyses of dry matter (drying at 125°C until constant weight), protein (Kjeldahl apparatus, % nitrogen × 6.25), and ash (incineration at 600°C for 6 hours) were performed for both diet and whole-body sample proximate composition analysis. Lipid content was quantified by the method described by Folch et al. (1957). AA content in the diet and whole-body samples were analyzed by reversed phase high-performance liquid chromatography according to the method described by Bartolomeo & Maisano (2006).
Data were subjected to one-way analysis of variance (ANOVA) in SPSS version 24.0 (IBM, Armonk, NY, USA). The differences among groups identified by ANOVA were compared using Duncan’s multiple range test (p < 0.05). A follow-up trend analysis by orthogonal polynomial contrast was run to determine whether the effect is linear and/or quadratic. All the data are presented as mean ± SD. The optimum dietary Thr requirement was analyzed by the broken-line regression analysis (Robbins, 1986).
Results
Weight gain (WG), specific growth rate (SGR), FI, feed conversation ratio (FCR), protein efficiency ratio (PER) and survival were significantly increased in fish fed dietary Thr levels over 0.8%; however, no significant changes were observed with gradual increases of Thr from 0.8% to 2.3% (Table 3). The lowest performance was exhibited by fish fed Thr0.4 diet (p < 0.05). There were significant linear and quadratic trends regarding the dietary Thr levels on final body weight, WG, SGR, FI, FCR, PER and survival. The optimum dietary Thr level for olive flounder was likely to be approximately 1.03% in diet based on the broken-line regression analysis (Fig. 1).
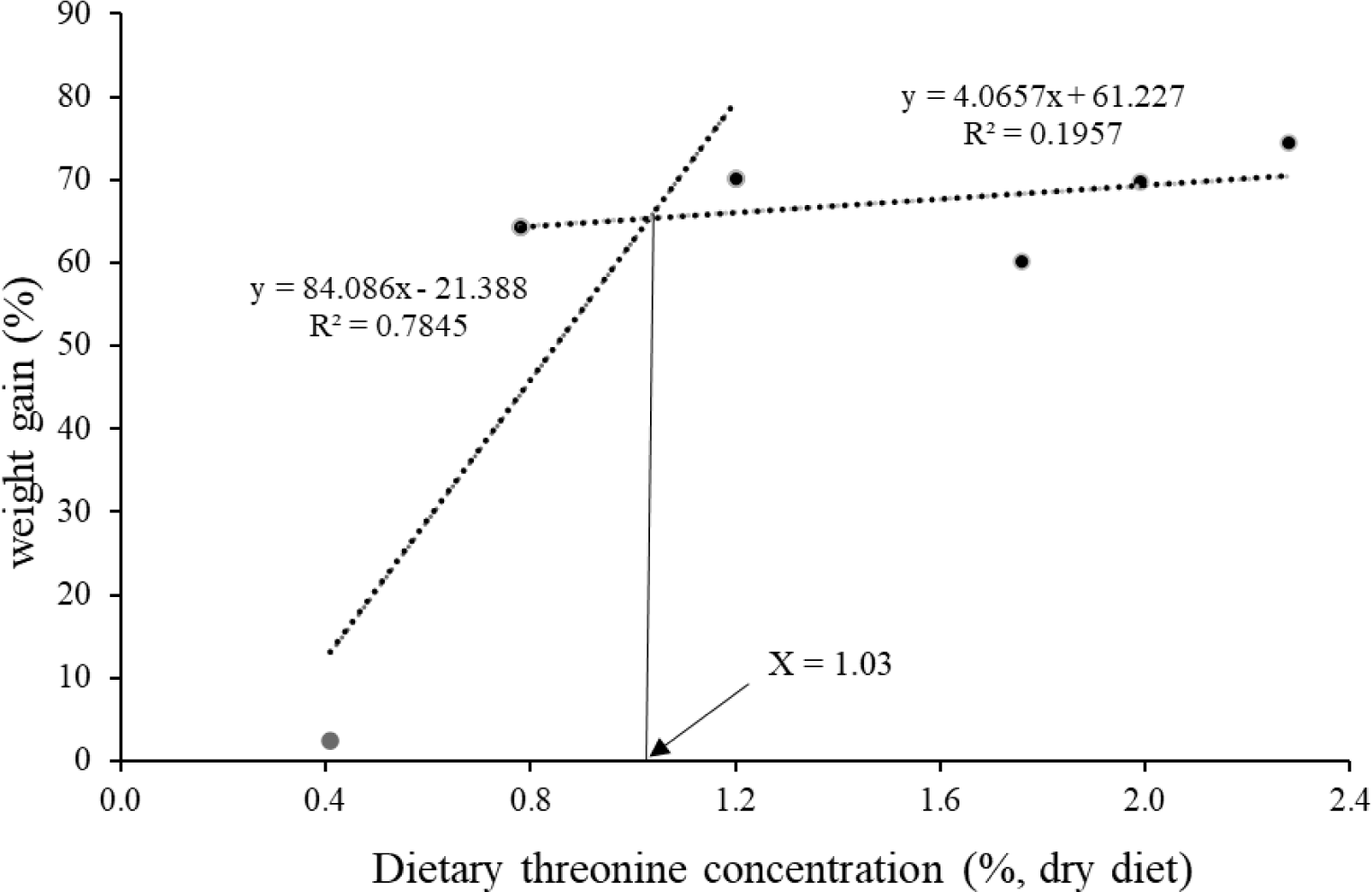
Non-specific immune parameters of lysozyme, MPO activity, antiprotease activity, and total immunoglobulin were significantly increased by dietary Thr levels by over 0.8% (Table 4). All the immune responses exhibited significant linear and quadratic trends regarding the dietary Thr levels.
Whole-body AA composition was not significantly affected by the Thr levels (Table 5). However, most EAAs tended to increase in fish fed Thr supplemented diets compared to those fed the basal diet. The growth of fish fed the basal diet hardly increased until eight weeks and thereafter drastically decreased (Fig. 2A). The survival of fish fed the basal diet also exhibited decreasing trend during the feeding trial (Fig. 2B).
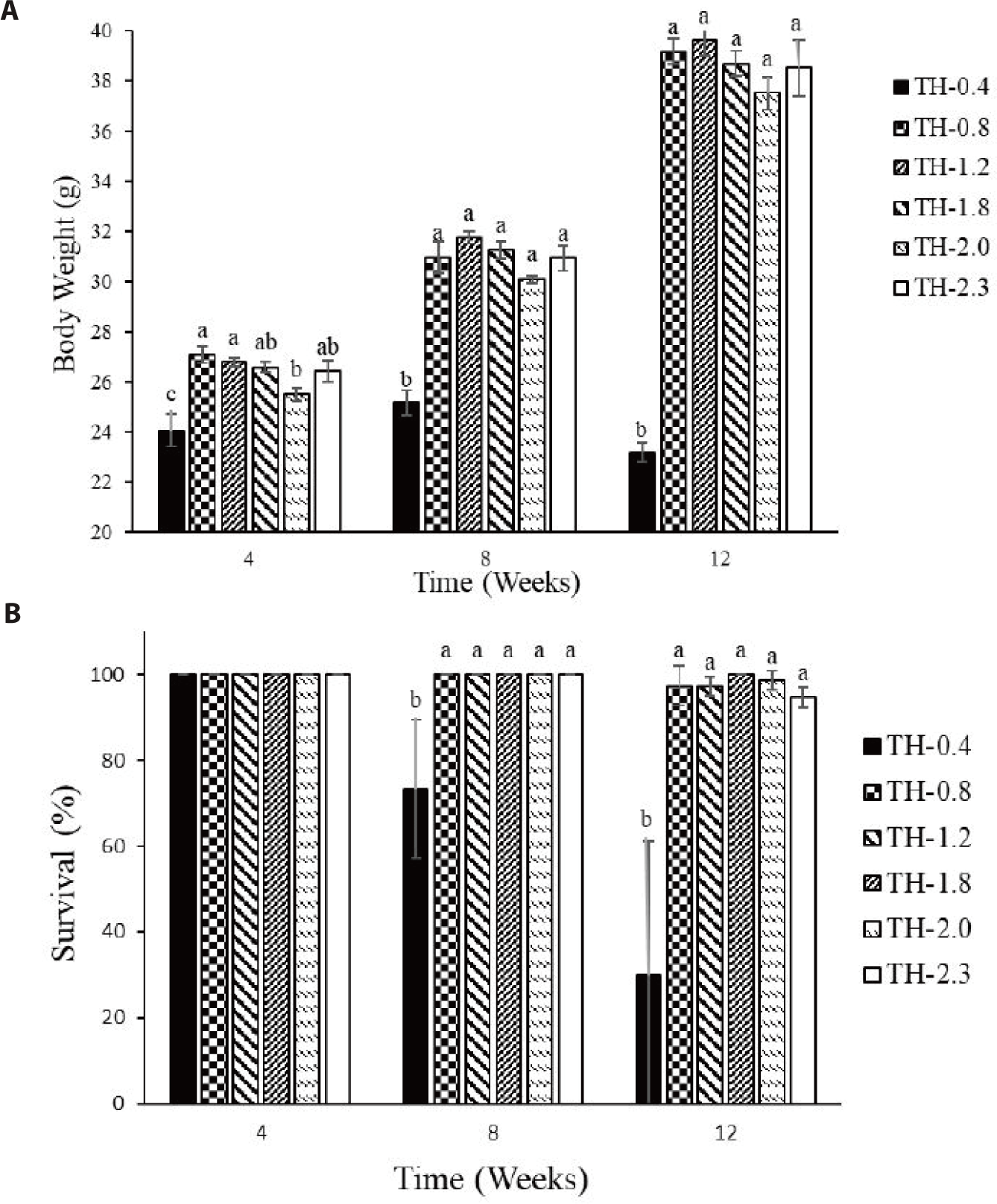
Discussion
This study demonstrates that Thr is an EAA, modulating growth performance, feed utilization and survival of olive flounder. Optimum dietary Thr requirement of several aquaculture species have been estimated in previous studies; Rainbow trout 1.1% (Bodin et al., 2008), Indian catfish 1.2% (Ahmed, 2007), Rohu carp 1.5% (Fatma Abidi & Khan, 2008), Nile tilapia 0.83% (Liebert, 2009), and Jian carp 1.6% (Feng et al., 2013) which are similar to olive flounder (1.03%) in this study. Thr exerts a key impact on physiological regulation, particularly in cell proliferation and growth (Tang et al., 2021). It is reported that Thr is a precursor for protein synthesis and a signaling molecule that can regulate the protein biosynthesis pathway (Lee & Li, 2014). Zhao et al. (2020) demonstrated that Thr upregulated MRFs (MyoD, Myf5, MyoG and Mrf4), which control the determination and differentiation of skeletal muscle cells and myosin heavy chains required for muscle growth via hypertrophy and hyperplasia of muscle fibers. Further, Zhao et al. (2020) observed that Thr upregulated the mRNA expression levels of TOR, an evolutionarily conserved serine/Thr kinase that regulates cell cycle progression, cell growth and protein synthesis (Seiliez et al., 2008). Thr is also known to regulate the PI3K/AKT signaling pathway, which directly links to myoblast differentiation and fish muscle protein synthesis (Fuentes et al., 2011). In the present study, whole-body AA composition was relatively decreased in the control group compared to Thr fed groups, indicating that Thr is required for the synthesis of body protein (Helland & Grisdale-Helland, 2006). However, despite their importance, the specific molecular mechanisms through which Thr modulates growth-related intracellular pathways in fish are poorly understood. Moreover, Thr is metabolized via Thr dehydrogenase to different intermediates and end products including pyruvate, acetyl CoA and glycine, which play essential roles in metabolism and energy production (Cheng et al., 2019; House et al., 2001).
In the present study, Thr deficient control group exhibited the lowest FI. Similarly, reduced FI was observed in Nile tilapia (Oreochromis niloticus) due to Thr deficiency (Liebert & Benkendorff, 2007). In response to Thr deficiency, reduced growth was reported due to anorectic effects in rohu carp (Labeo rohita) (Fatma Abidi & Khan, 2008), grass carp (Gao et al., 2014), blunt snout bream (Megalobrama amblycephala) (Habte-Tsion et al., 2015), and Indian catfish (Heteropneustes fossilis) (Ahmed, 2007). It has been demonstrated that fish reduce their FI when fed imbalanced EAA diets (Dabrowski et al., 2007; Gietzen et al., 2016; Gloaguen et al., 2012). Yamamoto et al. (2001) found that teleost fish such as rainbow trout (Oncorhynchus mykiss) exhibited an apparent aversion to EAA-deficiency diets. Dietary deficiencies of EAAs are detected by a specific brain region, anterior piriform cortex (APC) (Koehnle et al., 2004). The lower concentration of EAAs in APC may trigger a sensory signal that allows fish to recognize dietary AA deficiencies and mediate taste aversion (Gietzen, 1993). Therefore, the reduced FI observed in the control fish group in this study might be due to the EAA imbalance in the diet. Other potential olfactory responses and neurological responses of fish to EAA deficient protein sources have not been elucidated. A mechanism of how the EAA deficiency can cause an anorectic response in fish needs further study. According to the present study, it can be assumed that retarded growth in the control group might be partly due to the reduced FI.
Based on our results, dietary Thr significantly enhanced feed efficiency and protein utilization. Thr improved feed utilization efficiency in several fish species including Indian catfish (Ahmed, 2007), Pacific white shrimp (Zhou et al., 2013) and rohu carp (Fatma Abidi & Khan, 2008). Feed utilization efficiency is affected by digestion and absorptive capacity (Rønnestad et al., 2007). Thr is a primary component to secrete intestinal mucin that regulates gut barrier function, interacts with epithelium and microbiota and maintains intestinal homeostasis. Thr enhanced the digestive ability of Jian carp by increasing trypsin, lipase and amylase activities (Feng et al., 2013). Moreover, Thr increased enterocyte proliferation and differentiation and enhanced the activities of intestinal brush border enzymes such as Na+/K+–ATPase, γ glutamyl transpeptidase and alkaline phosphatase in Jian carp (Feng et al., 2013). Similarly, Thr deficiency was also reported to reduce intestinal function, villus height, crypt width and serosa thickness of juvenile grass carp (Gao et al., 2014).
In the present study, growth and feed utilization efficiency were comparatively lower than those reported in previous studies on olive flounder (Rahimnejad & Lee, 2014; Zhao et al., 2020). It might be due to the poor assimilation and utilization of crystalline AAs used as the primary protein source in formulated diets. Olive flounder is a typical carnivore marine flatfish species. Fish meal is the main protein source in commercial olive flounder feeds accounting for approximately 60% of the diet (Choi et al., 2020). Fish meal has a naturally balanced nutritional composition of protein, EAAs, energy, vitamins, minerals, long-chain polyunsaturated omega‐3 fatty acids and natural feed attractants (Hardy, 2010; Tacon & Metian, 2008). In the present study, because of the use of a crystalline AA mixture as the primary protein source, the AA utilizations might have been extremely low in the fish, which caused relatively low growth performance compared to those fed fish meal based diet.
The fish growth in the control group was remarkably lower throughout the experimental period and decreased drastically after eight weeks (Fig. 2A). The decreases in body weight might be linked to the consumption of body fat reserves for energy expenditure (Westerterp, 2010). The survival rate also exhibited a decreasing trend in the control group (Fig. 2B). The fish fed diets deficient in EAAs showed depressed immune responses and appeared more susceptible to infections in kidney, spleen, gills and skin (Konashi et al., 2000; Li et al., 2007). Thr regulates to secrete mucin, antimicrobial substance and immunoglobulins that are essential to immune regulation (McAuley et al., 2007). It is also reported that lymphocytes use Thr for proliferation and antibody secretion (Zhang et al., 2016). Habte-Tsion et al. (2015) observed that Thr increased white blood cells, red blood cells, immunoglobulin and antioxidant enzyme activities including superoxide dismutase, glutathione peroxidase and catalase in blunt snout bream. Dietary Thr deficiency resulted in low antibody levels and serum immunoglobulin concentrations in teleost fish (Uribe et al., 2011; Wilson et al., 1995). Further, Thr is known to modulate inflammatory cytokines and its deficiency increased intestinal inflammation, promoting pro-inflammatory cytokine tumor necrosis factor-α in blunt snout bream (Habte-Tsion et al., 2015). Thr deficiency is subjected to impair gill immune and its physical barrier function in grass carp (Dong et al., 2018). Thus, low survival rate and decreased immune response in the control group in this study might be due to the nutritional deficiencies, high susceptibility to diseases and pathological lesions. The mechanisms by which Thr mediates fish immune and antioxidant responses might be further characterized.
In conclusion, this study demonstrates that the dietary Thr requirement for the optimum growth and immune response in olive flounder is likely to be 1.03% in the diet. The deficiency of dietary Thr significantly attenuates the growth, feed intake, feed utilization, innate immunity and survival of fish.