Introduction
Scuticociliatosis is the prevalent parasitic disease affecting finfish aquaculture worldwide. It is caused by various ciliate species, including Uronema nigricans, Philasterides dicentrarchi, Uronema marinum, Pseudocohnilembus persalinus, and Miamiensis avidus. Among these species, M. avidus is the primary agent responsible for scuticociliatosis in olive flounder (Paralichthys olivaceus) aquaculture (Kang & Kim, 2014; Song et al., 2009a). Olive flounder infected with M. avidus suffer from severe systemic infections, leading to organ-specific pathological changes from the skin to the brain (Kim et al., 2019). Moreover, M. avidus infection also increases the risk of secondary bacterial diseases, such as tenacibaculosis and vibriosis (Jung et al., 2007). Current control measures for scuticociliatosis include antibiotics and formalin treatments, although their efficacy is limited to the cases of mild infection (Iglesias et al., 2002; Jee & Jo, 2002). Furthermore, the government strictly regulates their usage due to concerns related to food safety and environmental impact. Therefore, immunization of fish with an effective vaccine against M. avidus infection is crucial for successfully preventing scuticociliatosis without compromising public health. Researchers have developed experimental vaccines that show promising preventive effects against scuticociliatosis under laboratory conditions (Lamas et al., 2008; León-Rodríguez et al., 2012). Nevertheless, there is a lack of comprehensive field data on the efficacy and safety of these vaccines.
In this study, we conducted a 5-year-investigation into the emergence pattern of M. avidus and the co-infecting bacteria, Tenacibaculum maritimum, in 20 olive flounder farms on Jeju island, South Korea. Through comprehensive disease monitoring, including M. avidus serotyping and analysis of T. maritimum co-infection, we developed a trivalent vaccine containing two different serotypes of M. avidus and one strain of T. maritimum to prevent the corresponding diseases in farmed olive flounder. The vaccine’s efficacy has been assessed through experimental infection under laboratory conditions and further validated through a field test conducted on different fish farms.
Materials and Methods
The intensive monitoring was implemented at 20 different olive flounder farms located at Seongsan, Pyoseon, Namwon, Daejeong, Hangyeong, Jocheon, and Gujwa in Jeju island, Korea (Fig. 1A). Five fish were randomly collected from each farm every month. In total, 6,200 olive flounder samples were obtained between February 2015 and March 2020. The collected fish were examined using a light microscope to determine if infection by M. avidus was present.
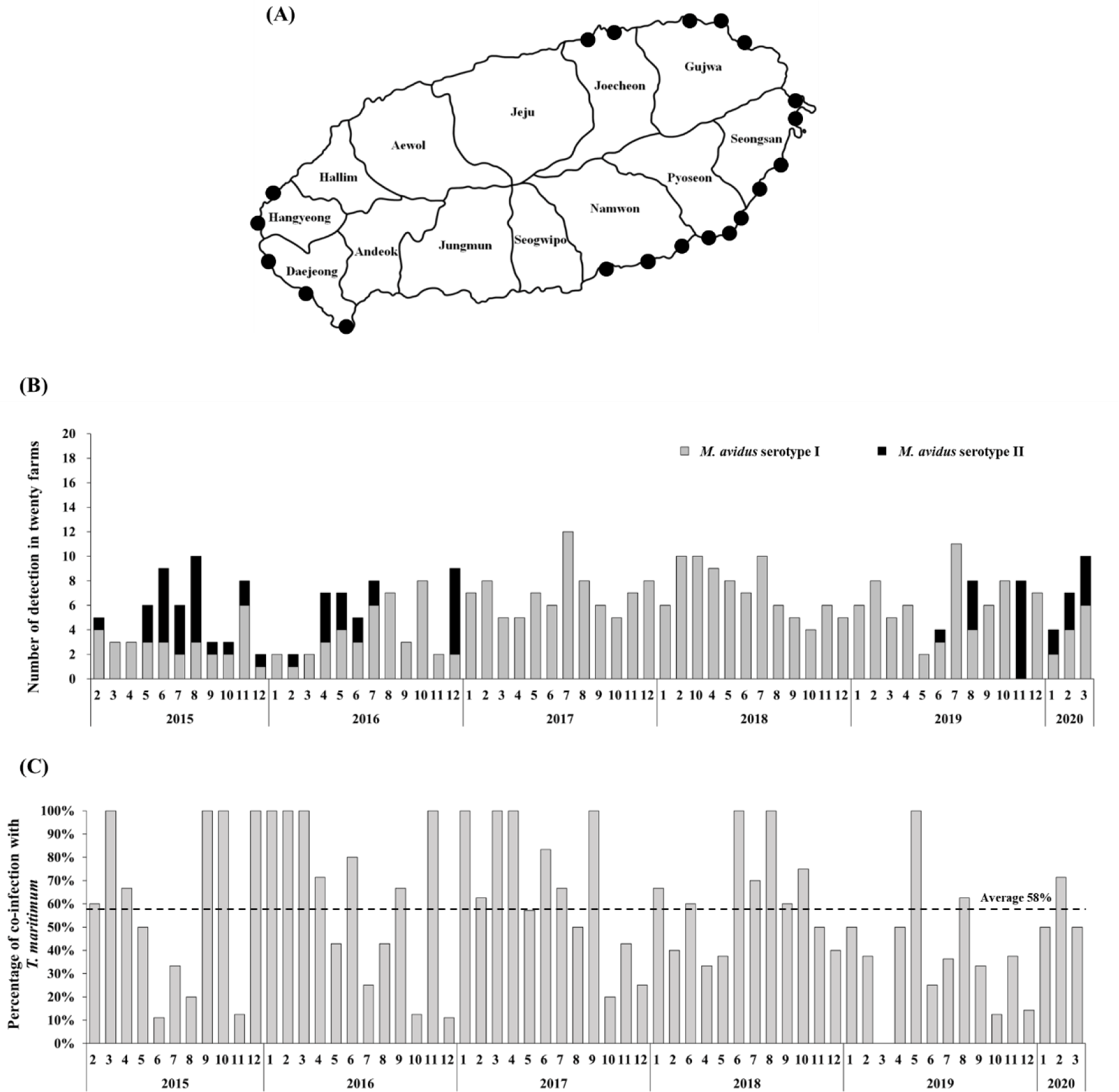
T. maritimum was isolated from the liver, kidney, and spleen of olive flounder suffering from scuticociliatosis and incubated on DifcoTM marine agar plates (BD, Franklin Lakes, NJ, USA). Following 48 hr-incubation at 25°C, the isolated strains were confirmed by microscopic and molecular analysis using polymerase chain reaction (PCR) with species-specific primers (forward: 5′-AATGGCATCGTTTTAAA-3′, reverse: 5′-CGCTCTCTGTTGCCAGA-3′; Avendaño-Herrera et al., 2004).
M. avidus strains isolated from the infected fish were cultured by feeding the parasite with fathead minnow (FHM) cells. Genomic DNA was extracted using DNeasy Blood & Tissue kit (Qiagen, Hilden, Germany). The PCR was performed to amplify the mitochondrial cytochrome c oxidase subunit 1 (cox1) genes of M. avidus using the specific primers (cox1 forward: 5′-GGTTCTAAAGATGTGGCTTACCCTAGAC-3′, cox1 reverse: 5′-CATACCAGGCATACATAAAGTACGTCTTGT-3′). The amplified PCR fragment was purified, cloned into the pMD20-T vector, and sent for sequencing (Macrogen, Seoul, Korea). The obtained cox1 nucleotide sequences of M. avidus were aligned with those reported in previous studies using ClustalW (http://www.ebi.ac.uk/Tools/msa/clustalw2/). A phylogenetic tree was constructed using the neighbor-joining method using the cox1 sequences. The percentage of replicates was 1,000 replicates.
Healthy olive flounders with an average body weight of 8 g were purchased from a local farm in Jeju island, South Korea. The fish were first diagnosed as free of pathogen infection and acclimated to experimental conditions at 20°C for one week before the initiation of the experiment. The M. avidus parasite was cultured by feeding with FHM, which were grown in Leibovitz’s L-15 (L-15) medium containing 100 U/mL penicillin and 100 µg/mL streptomycin supplemented with 10% fetal bovine serum at 20°C, as described by Dyková et al. (2010). The harvest of M. avidus was done by centrifugation at 500×g for 15 min at room temperature, and the resuspended cells were counted by hemocytometer under a light microscope. The T. maritimum bacteria were cultured in a marine broth medium at 25°C for 24 h. The grown bacteria were centrifuged at 4,000×g for 30 min and resuspended in phosphate-buffered saline (PBS). The T. maritimum cell number was determined using optical density measurements (OD) at a wavelength of 600 nm and the equation of 1OD = 2 × 108 CFU.
A group of 20 fish were injected intraperitoneally (IP) with 100 µL of varying cell concentrations (1 × 104, 1 × 105, and 1 × 106 cells/fish) for M. avidus JJB1403 and JJC1404 strains, respectively. Similarly, the T. maritimum KOR-JJ strain was IP injected into fish at concentrations of 1 × 107, 1 × 108 and 1 × 109 CFU/fish. Fish injected with the same volumes of L-15 medium or PBS solution were used as negative controls. Injected fish were placed in 70-L tanks filled with 50-L aerated seawater treated by UV and filtered through 1-micron cartridge filters. Water change was done twice daily, and the mortalities were recorded daily for 21 days. Ethical clearance for animal experiments was obtained from the Institutional Animal Care and Use Committee of Jeju National University, Republic of Korea (Approval no. 2018-0005).
A trivalent vaccine was prepared using cells from the M. avidus JJB1403, JJB1404, and T. maritimum KOR-JJ strains that had been inactivated using formalin. M. avidus and T. maritimum were cultured using the method described in the virulence evaluation experiment above. The formalin fixation of M. avidus was performed using a final concentration of 0.3% formalin with continuous stirring for 1 h at 25°C, and T. maritimum was treated with 1% formalin for 72 h at 4°C. The adjuvant was prepared with 25% squalene (Sigma, S3626), 2.5% Tween 80 (Sigma, P1754), and 2.5% Span 85 (Sigma, S7135) in PBS via microfluidization at 12,000 psi as described in a previous study (Calabro et al., 2013). Finally, the M. avidus JJB 1403 (2.5 × 106 cells/mL), M. avidus JJC 1404 (2.5 × 106 cells/mL), T. maritimum KOR-JJ (2 × 109 CFU/mL) strains and 10% adjuvant were homogenized using a high shear mixer at a speed of 8,000 rpm for 30 min.
Healthy olive flounders with an average body weight of 15 g were purchased from a local olive flounder farm in Jeju island, Korea. Fish were cultured in three 2,000 L flow-through seawater tanks (n = 150 fish/group with three groups), with a 1,000% daily water exchange and continuous aeration at 18°C. After one week of acclimation, 100 µL of trivalent vaccine, adjuvant, and PBS were IP injected into the fish in each group, respectively. At four weeks post-vaccination, each experimental group (n = 20 fish/tank) was injected with 5 × 105 cells/fish of M. avidus (JJB1403, JJC1404) and 8 × 107 CFU/fish of T. maritimum. The mortalities were recorded for 21 days, and the efficacy of the vaccine was evaluated according to the formula below.
Ten fish were sampled from the experimental groups at zero, two, four, six, and eight weeks post-vaccination. Blood was collected from the caudal vein of the olive flounders from each experimental group. Blood samples were kept at room temperature for 1 h, stored overnight at 4°C, and then centrifuged at 3,000×g for 10 min at 4°C to obtain the serum. Serum samples were stored at –20°C until use.
96-well flat-bottom culture plates were coated at 100 µL per well with M. avidus JJB1403 and JJC1404 (1 × 106 cells/mL) and T. maritimum (1 × 109 CFU/mL), along with a coating buffer (30 mM Na2CO3 and 70 mM NaHCO3 at pH 9.0). The plates were incubated for 24 h at 4°C. After washing with tris buffered saline (TBS) containing 0.1% Tween 20 (TBS-T; 1%), plates were blocked with 200 μL skim milk solution (TBS-T with 5% skim milk) for 1 h at 4°C. Plates were then rinsed with TBS-T solution, and the wells were treated with serum (90 μL skim milk solution with 10 μL serum). After a 2 h incubation period at 4°C, the plates were washed three times with TBS-T solution and incubated with 100 μL of mouse anti-flounder IgM (1: 1,500) for 2 h at 4°C. Plates were rinsed again with TBS-T solution, and 100 μL horseradish peroxidase (1: 1,500) conjugated goat anti-mouse secondary antibody (YOUNG IN Frontier, Seoul, Korea) was added. After 2 h of incubation at 4°C, the plates were washed three times with TBS-T solution. Finally, 3,3′,5,5′-Tetramethylbenzidine liquid solution (Sigma-Aldrich, St. Louis, MO, USA) was added. The reaction was stopped with 2 N H2SO4, and the absorbance was measured at 450 nm using a microplate spectrophotometer (Multiskan GO, Thermo Fisher Scientific, Waltham, MA, USA).
Four olive flounder farms labeled A, B, C, and D, were selected to evaluate the efficacy of the vaccine under field conditions. The number of fish that were vaccinated is described in Table 1. Fish were subjected to fasting two days before vaccination. Following the fasting period, 0.1 mL of trivalent vaccine was IP injected into fish with an average size of 42.5 g at farm A, 65.2 g at farm B, 55.3 g at farm C, and 52.5 g at farm D, respectively. Mortality in each group was recorded daily. Dead fish were collected, and scrapes of the gills and skin were examined via microscopy to determine the level of infection by M. avidus and T. maritimum. Furthermore, bacterial strains were isolated from liver, kidney, and spleen smears and incubated on marine agar plates at 25°C for 48 hours. The cultured bacteria were identified using T. maritimum specific PCR primers. Ethical clearance for animal experiments was obtained from the Institutional Animal Care and Use Committee of Jeju National University, Republic of Korea (Approval no. 2018-0006).
Results
During the period from 2015 to 2020, a total of 390 M. avidus infections were identified. Serotyping analysis revealed the presence of two serotypes of M. avidus (serotype I and serotype II) on Jeju island, accounting for 83% and 17% of the total identified M. avidus infections, respectively (Fig. 1B). Notably, during the investigation, it was found that more than 50% of the M. avidus infections were accompanied by T. maritimum co-infections.
Sequence analysis revealed that M. avidus serotype I and II possess distinct sequence variations in the cox1 gene at 16 loci (Fig. 2). For phylogenetic analysis, the nucleotide sequences of the cox1 gene from M. avidus serotype I (M. avidus JJB1403) and M. avidus serotype II (M. avidus JJC1404) were compared with the cox1 sequences reported in previous studies (Jung et al., 2011). The results indicated that M. avidus JJB1403 was clustered together with 11 other strains (Nakajima, GJ01, WDS-0709, WDB-0708, SJF-06A, YS2, WD4, JJ4, JJ3, WS1, and SJF-03A) as serotype I, whereas M. avidus JJC1404 was clustered with seven other strains (SJF-03B, YK1, YK2, JF05To, RF05To, SK05Kyo, and Iyo1), confirming its classification as serotype II (Fig. 3).
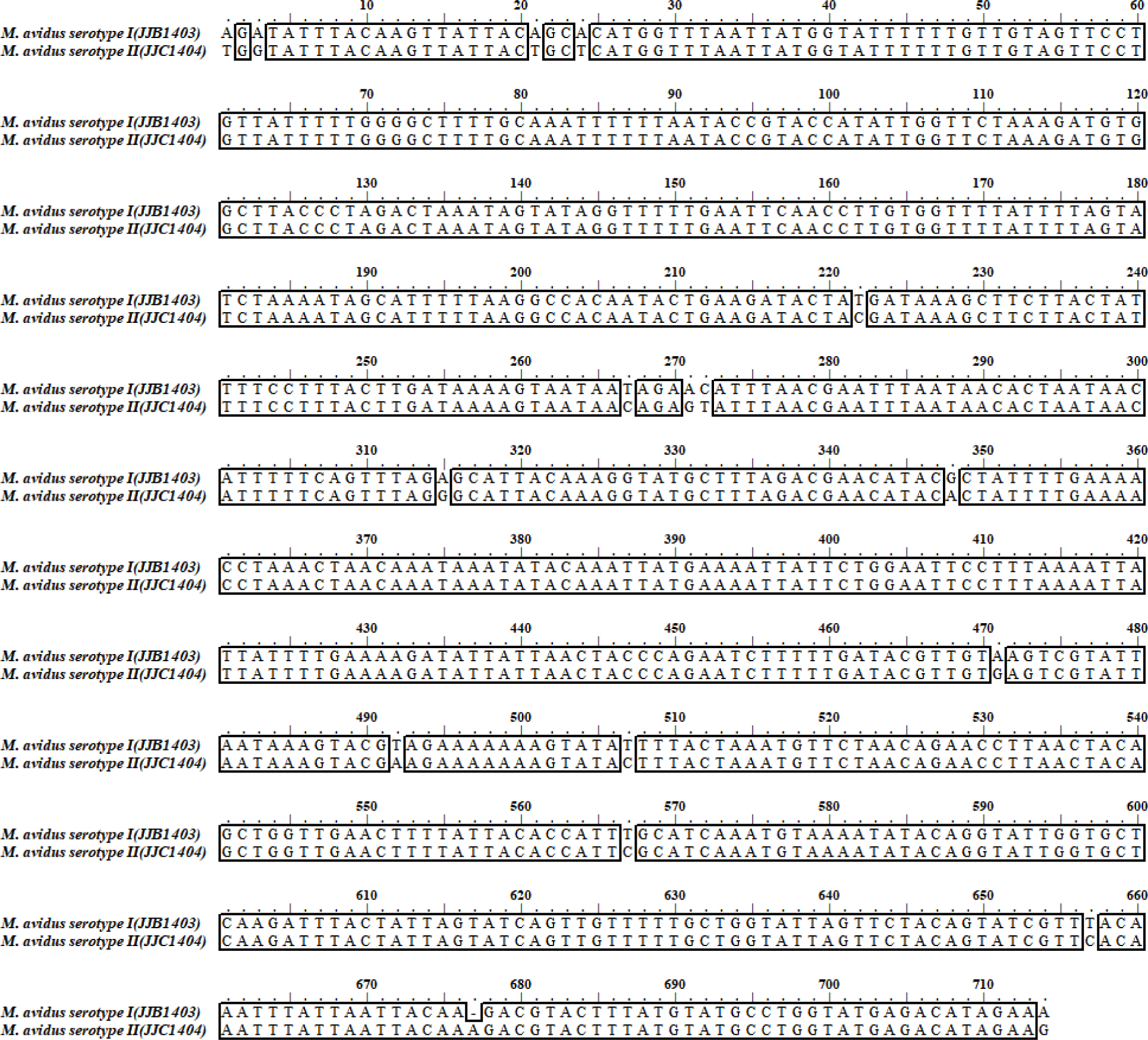
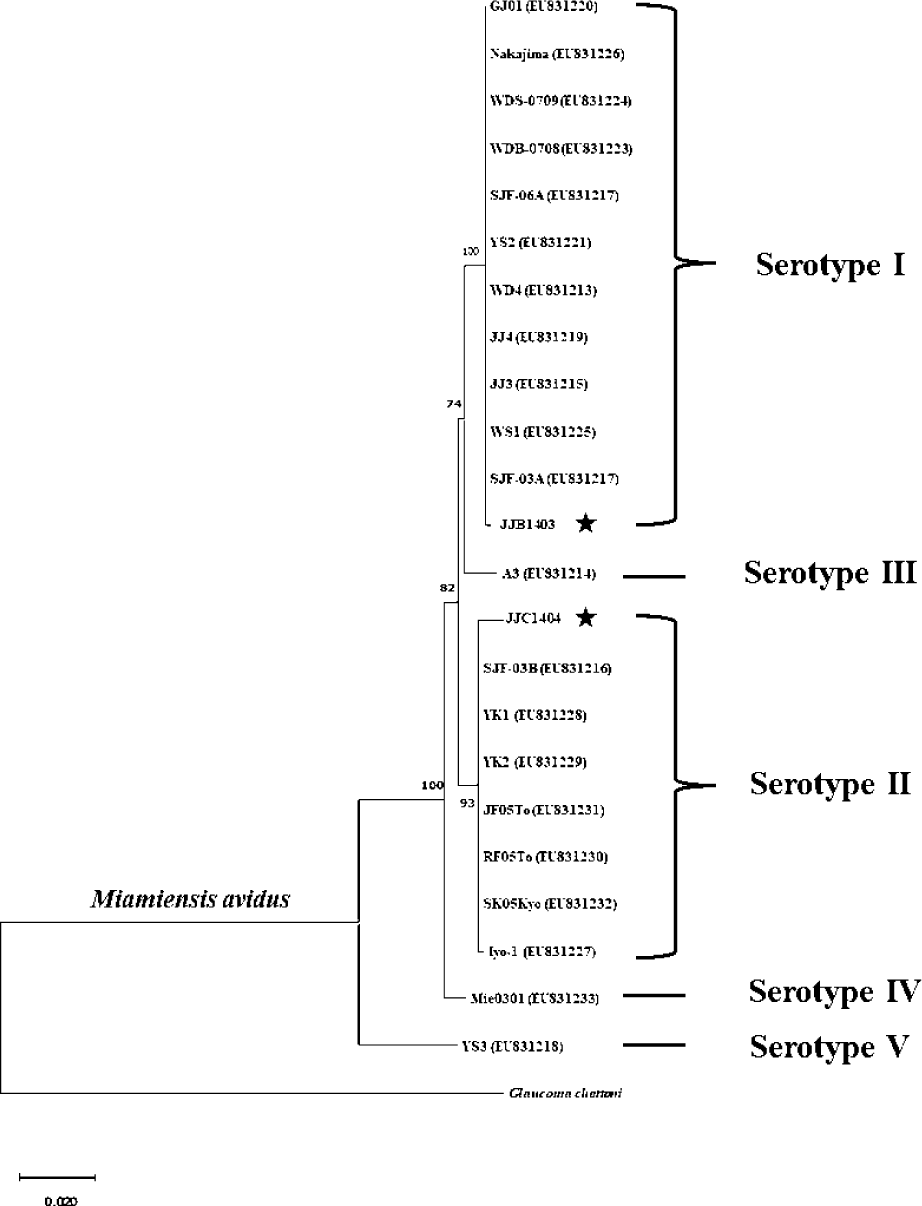
In the virulence test, the cumulative mortality of M. avidus JJB1403 and M. avidus JJC1404 exceeded 70% across all three dosage levels (Fig. 4A and 4B). For T. maritimum KOR-JJ, fish injected with a concentration of 1× 109 cells/fish exhibited 100% mortality within five days post-injection. At concentrations of 1 × 108 cells/fish and 1 × 107 cells/fish, the mortalities caused by T. maritimum KOR-JJ reached 55% and 30%, respectively (Fig. 4C). These results collectively demonstrate that the collected pathogen strains are virulent and pathogenic to olive flounder, making them suitable for vaccine development.
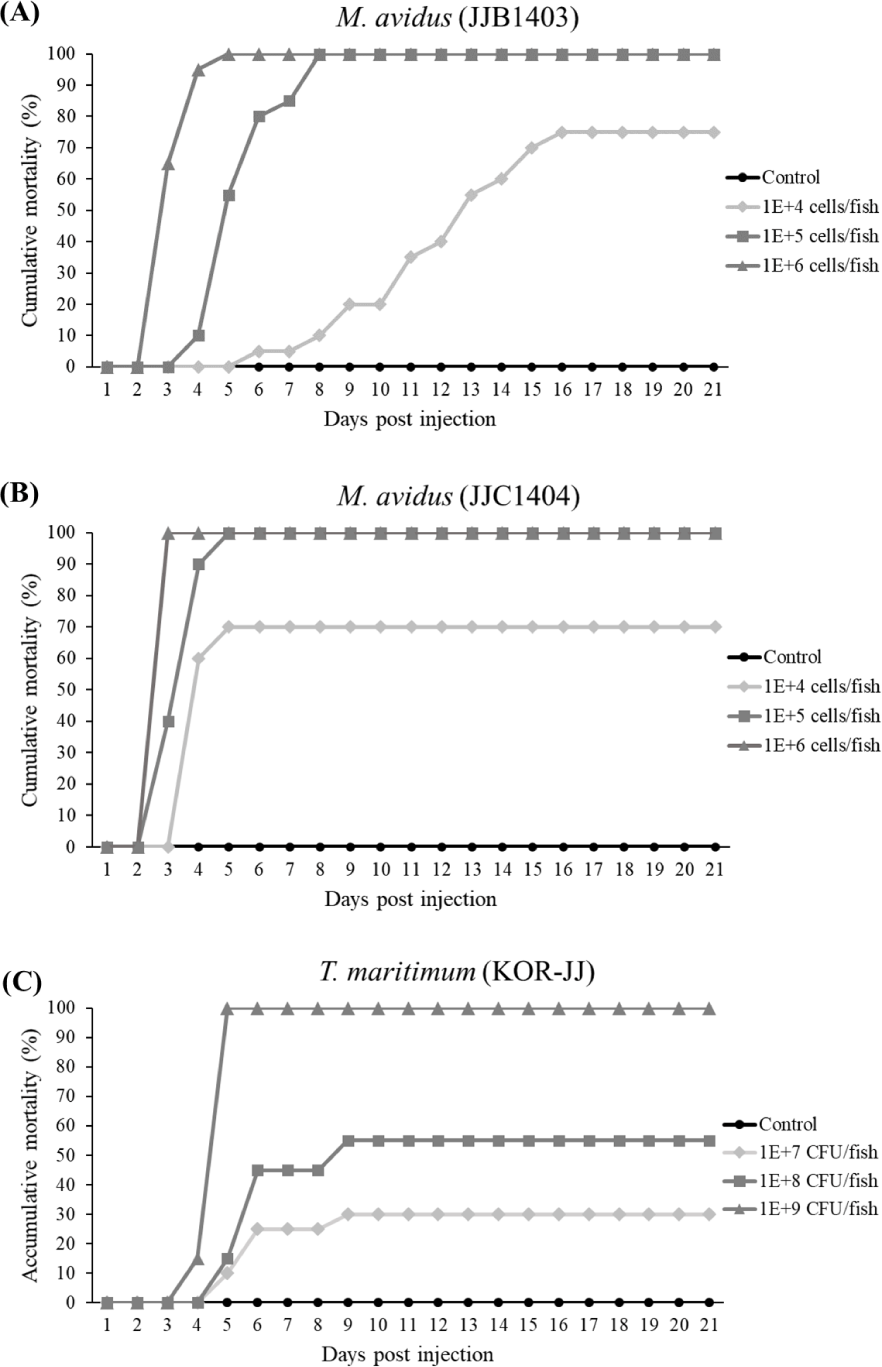
The final survival rates of the control group infected with M. avidus JJB1403, M. avidus JJC1404, and T. maritimum KOR-JJ were 20%, 15%, and 20%, respectively. In contrast, the vaccinated group showed significantly higher survivals, with rates of 80%, 75%, and 95% for M. avidus JJB1403, M. avidus JJC1404, and T. maritimum KOR-JJ, respectively. The group injected only with adjuvant exhibited a 20% survival rate after experimental infection with the three different strains (Fig. 5). The calculated RPS for the vaccinated groups were 75%, 80%, and 93% for M. avidus JJB1403, M. avidus JJC1404, and T. maritimum KOR-JJ, respectively (Fig. 5).
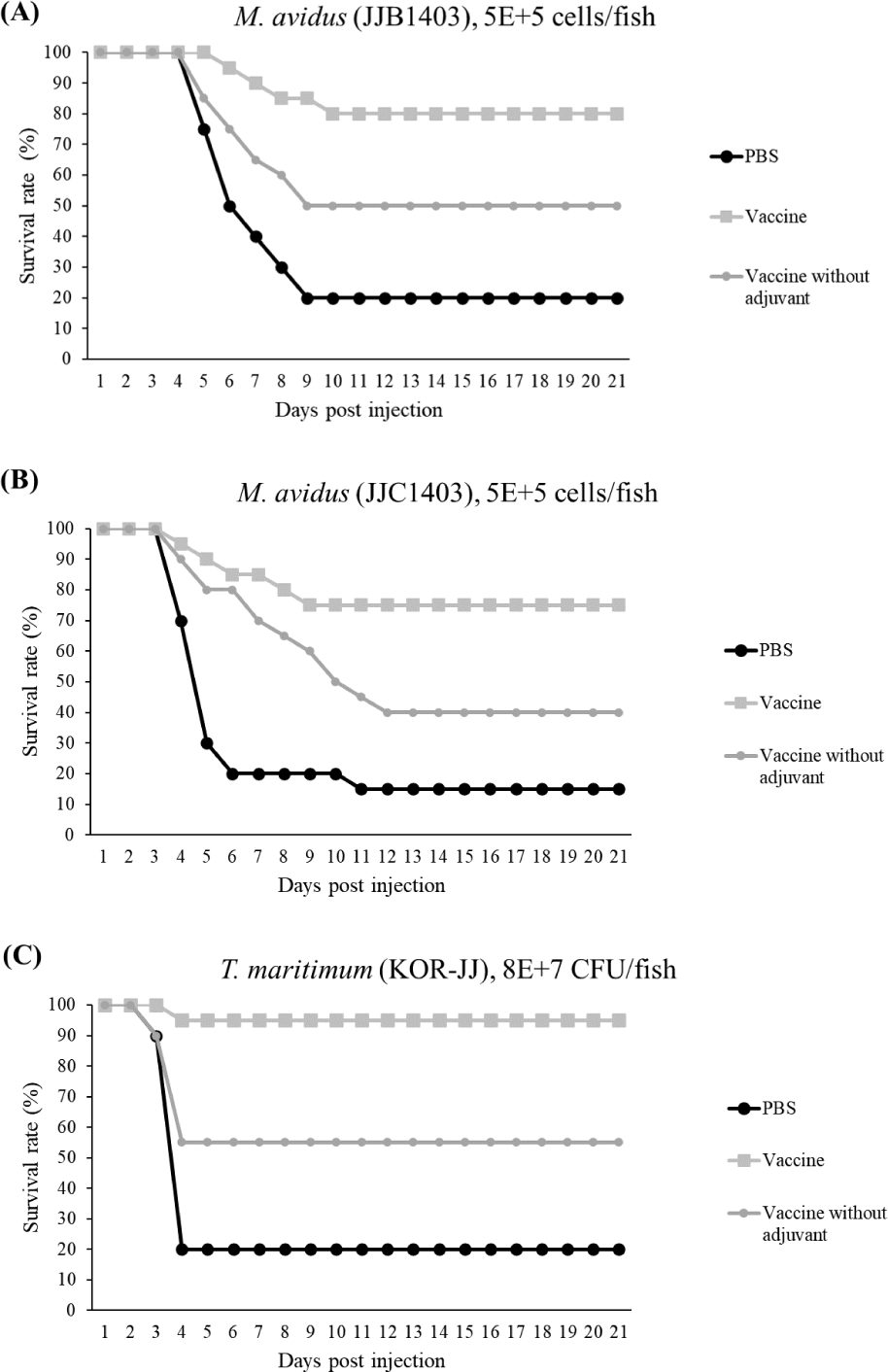
The vaccinated groups consistently exhibited higher levels of antibody compared to the control group against all three pathogens (Fig. 6). In the vaccinated group, antibody titers against M. avidus JJB1403, M. avidus JJC1404, and T. maritimum KOR-JJ steadily increased until four weeks post-vaccination (wpv) and subsequently began to decrease after six wpv. On the other hand, the antibody titers of the control group were maintained at a low level, showing no significant difference throughout the experiment.
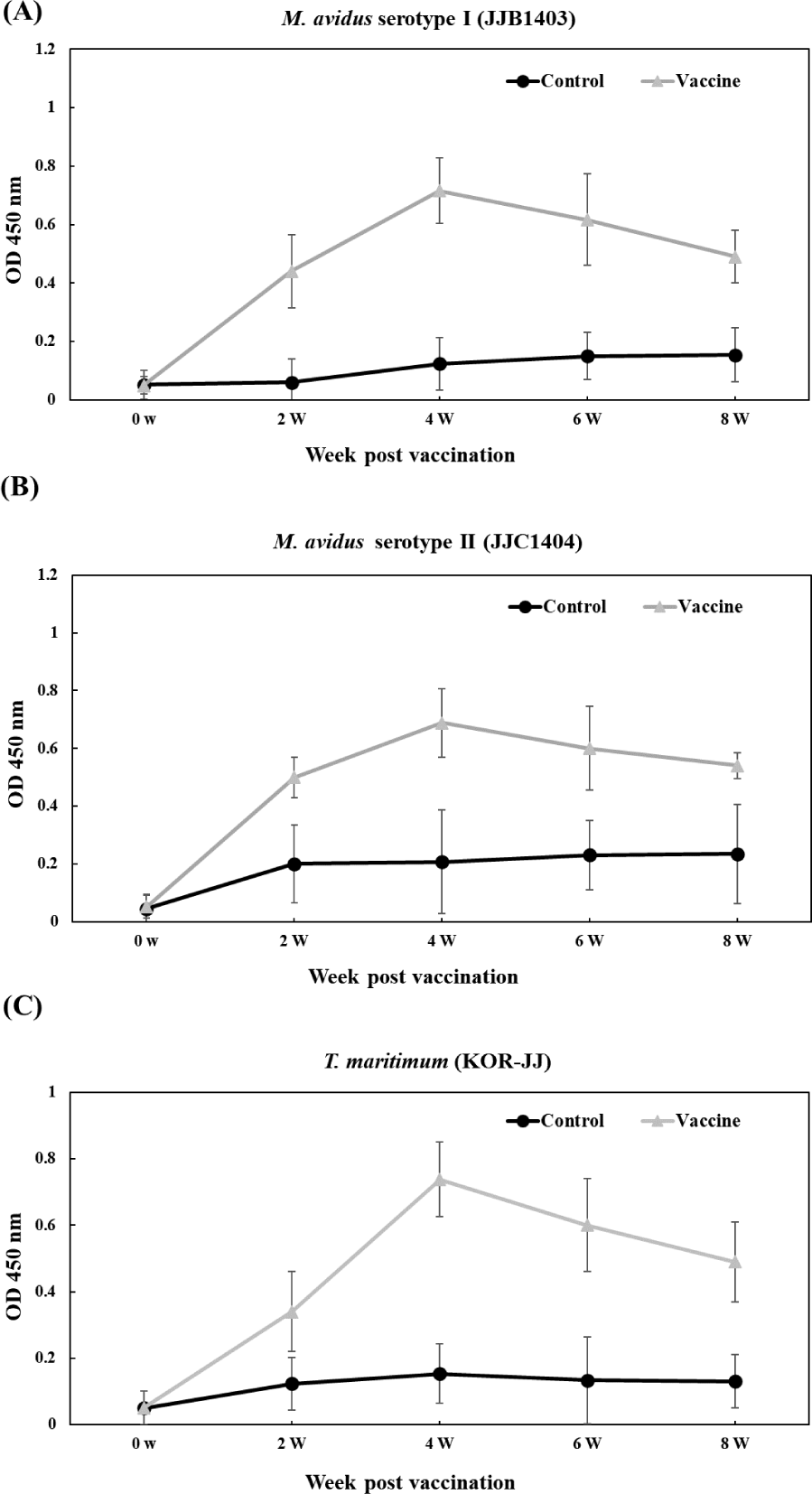
The efficacy of the trivalent vaccine for olive flounder was evaluated at four farms (Table 1). On farm A, the vaccinated group had 499 dead fish compared to 2,256 in the control group. The control group experienced a substantial increase in mortality starting at 4 wpv, while the vaccinated group showed a delayed onset of significant mortality and significantly lower rates of weekly and cumulative mortality (Fig. 7A). On farm B, the vaccinated group had 2,468 dead fish compared to 6,920 dead fish in the control group. Both groups experienced mortality due to M. avidus and/or T. maritimum infections, but the severity of the disease outbreak was significantly lower in the vaccinated group (Fig. 7B). On farm C, the vaccinated group had 317 dead fish, and the control group had 1,301 dead fish diagnosed with M. avidus and/or T. maritimum infections. The control group showed a sudden increase in mortality starting at 9 weeks post-vaccination, while the vaccinated group maintained consistently low weekly mortality throughout the experiment (Fig. 7C). On farm D, the vaccinated group had 1,262 dead fish, and the control group had 4,361 dead fish. Both groups had considerable mortality between 4 and 6 weeks post-vaccination, but the vaccinated group had significantly lower mortality (Fig. 7D). Overall, the developed vaccine demonstrated a significant reduction in mortality caused by M. avidus and/or T. maritimum infections compared to the control group, with RPS values ranging from 52.98% to 99.70% across the four farms.
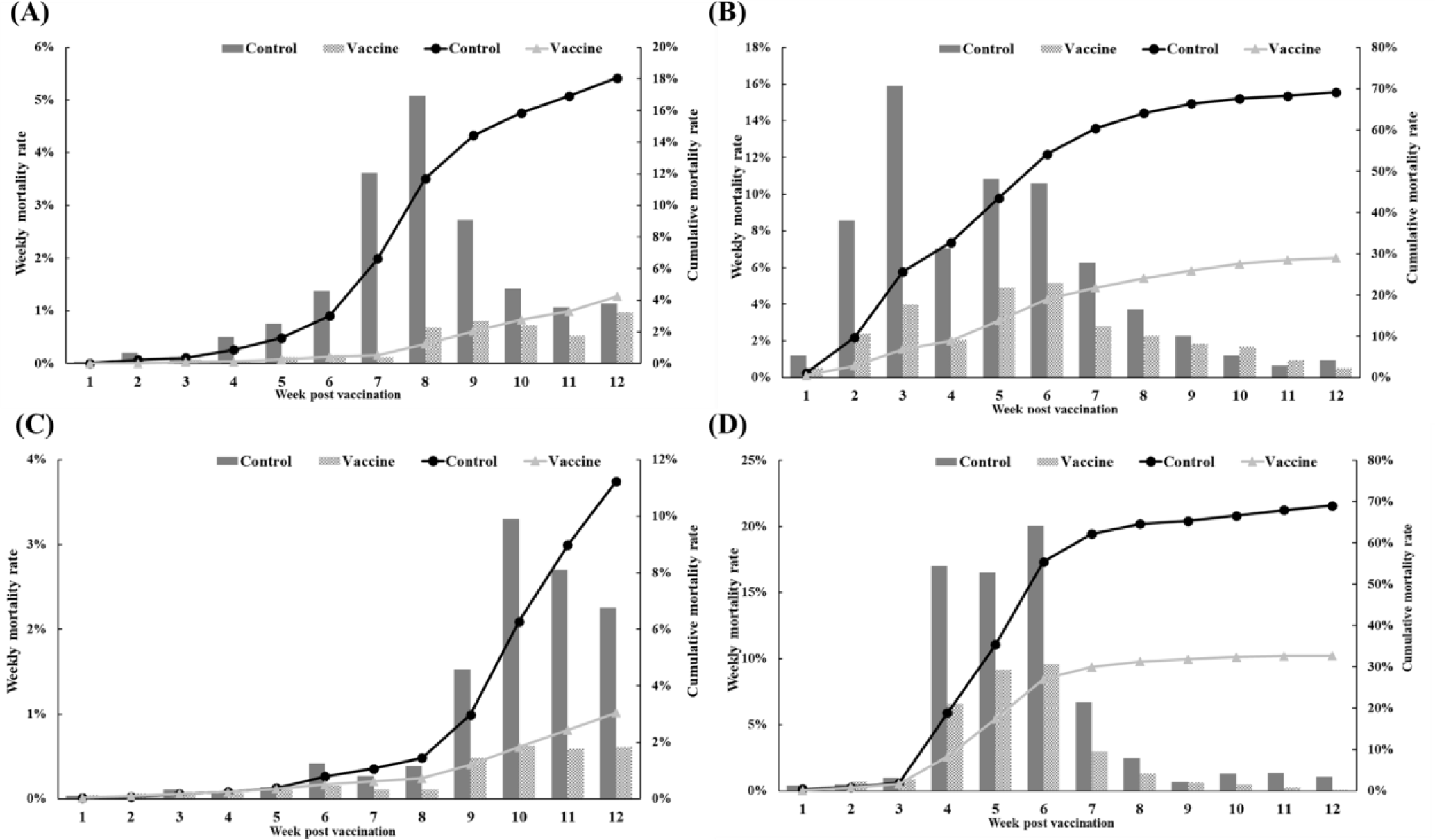
Discussion
The monitoring results revealed the presence of two serotypes of M. avidus on Jeju island as determined by cox1 gene sequence analysis. The cox1 gene has been widely recognized as a useful marker for species identification of animals due to its easy isolation, significant variation among species, minimal differences within species, and absence of introns (Rodrigues et al., 2017). Moreover, cox1 sequence analysis has been applied to the classification of ciliates to expand the utility of this technique within the phylum (Whang et al., 2013). Through comparison of the cox1 gene sequences, 21 M. avidus strains isolated from olive flounder (P. olivaceus), ridged-eye flounder (Pleuronichthys cornutus), and spotted knifejaw (Oplegnathus fasciatus) in Korea and Japan were classified into five serotypes (cox1 serotype I to V; Jung et al., 2011). Similarly, another study on M. avidus isolation during outbreaks of scuticociliatosis in Japan identified six isolates from different fish species and classified them into three serotypes through serological analysis (Song et al., 2009b). During the period between 2015 and 2020, we isolated a total of 390 M. avidus isolates from the disease olive flounder. Among them, serotype I (JJB1403) and serotype II (JJC1404) accounted for 83% and 17% of the total M. avidus isolates, respectively, indicating the new serotype distribution of M. avidus in Korean olive flounder aquaculture. There is a lack of research on the seasonal variation of M. avidus serotypes. Therefore, a specific investigation is warranted to understand these changes more comprehensively.
T. maritimum is the major causative agent of tenacibaculosis, the most frequently occurring secondary infection following the onset of scuticociliatosis in olive flounder (Jin et al., 2007). Our study observed an average co-infection ratio of 58% for M. avidus and T. maritimum in scuticociliatosis-affected olive flounder in the fish farms on Jeju island. Olive flounder infected with T. maritimum alone can be diagnosed by the presence of annular (ring-shaped) epidermal ulcerative lesions with dark-colored outer edges on the skin, along with frayed fins and a rotten tail (Mabrok et al., 2023). However, co-infection with M. avidus and T. maritimum leads to clinical signs closely resembling scuticociliatosis, characterized by multiple epidermal and dermal necrotic lesions on the skin and fins, which can extend deeply into the underlying muscles and cause severe inflammation at advanced infection stages (Moustafa et al., 2010). Co-infections between bacterial pathogens, viruses, and parasites are frequent in olive flounder aquaculture. However, due to the difficulty of distinguishing clinical signs, farmers often under-report or neglect the co-infections of T. maritimum during scuticociliatosis outbreaks, leading to a lack of associated information. Previous studies in other fish species indicate that the co-infection of bacterial pathogens may exert an immunosuppressive effect, which can potentiate the parasitic infection and significantly increase mortality (Wise et al., 2021). In our study, we developed a vaccine comprising two serotypes of M. avidus and T. maritimum, shedding light on its effectiveness in preventing sucuticociliatosis, especially in reducing co-infected bacterial disease burden and improving fish survival rates.
To enhance the vaccine efficacy, we employed a squalene-based emulsion (SE) adjuvant in the trivalent vaccine developed in this study. SE adjuvants, such as MF59, AS03, and AF03, are oil-in-water emulsions containing completely metabolizable oil droplets and surfactants. These adjuvants have been extensively studied and proven safe and effective, having received approval for human vaccine development in many countries (Wilson-Welder et al., 2009). The mechanism of SE adjuvant action is associated with promoting antigen uptake and recruiting immune cells, which leads to a stronger and more sustained activation of both the innate and adaptive immune systems (Nguyen-Contant et al., 2021). By incorporating the SE adjuvant, the trivalent vaccine developed in this study exhibited high immunogenicity, providing robust and long-lasting protection against scuticociliates and T. maritimum. Notably, several studies have also assessed vaccine efficacy and long-term effects associated with using oil-based adjuvants in fish. For instance, in turbot, vaccination with an antigen and mineral oil-based adjuvant resulted in significant protection against P. dicentrarchi and elicited high serum antibody titers (Sanmartín et al., 2008). Another study by Rahman et al. (2000) demonstrated that using a squalene adjuvant provided better protection than administrating the formalin-inactivated flavobacterium alone. Moreover, in olive flounder, a recent study evaluated the efficacy of the viral hemorrhagic septicemia virus (VHSV) vaccine and confirmed that adding a squalene adjuvant could increase both the efficacy and persistence of the vaccine (Vinay et al., 2013).
Through natural infections or immunization by vaccines, fish can generate specific antibodies against scuticociliates, which are directly associated with the defense against scuticociliatosis in various fish species (Piazzon et al., 2014). These antibodies agglutinate the ciliate cells to limit migration and exhibit direct cytolytic activity by activating the classical complement pathway (Palenzuela et al., 2009; Piazzon et al., 2014). Our study observed high antibody production in vaccinated fish throughout an eight-week experimental period, contributing to a high RPS during the scuticociliate challenge. Previous studies evaluated antibody levels after vaccination towards different scuticociliate strains in different fish species. For instance, in turbot, fish immunized with formalin-killed P. dicentrarchi vaccines produced significantly higher levels of antibody in sera compared to the non-vaccinated group, which maintained the ciliate agglutinating activity for up to 6 months post-vaccination (Iglesias et al., 2003; Sanmartín et al., 2008). Moreover, several studies have demonstrated good correlations between the levels of specific antibody production and the survival rate under scuticciliatosis in immunized fish through experimental challenge tests in several studies (Lamas et al., 2008). The use of adjuvants, such as mineral oil and non-mineral Montanide ISA 763A, has been reported to be crucial for increasing specific antibody production in scuticociliate vaccines (Lamas et al., 2008; Mabrok et al., 2023). Additionally, in a study carried out in kelp grouper, encapsulation of U. marinum antigen with poly D, L-lactide-co-glycolic acid (PLGA) showed a promoting effect on the specific antibody production at 2 weeks and 4 weeks post-vaccination (Harikrishnan et al., 2012).
In previous studies, several scuticociliate vaccine trials have been performed solely under laboratory conditions, showing protective potency against various ciliate stains, including P. dicentrarchi, U. marinum, and M. avidus. In olive flounder, three different types of vaccines (injectable, immersion, and oral) have been developed against M. avidus, resulting in RPS values of over 60% (Jung & Jung, 2021; Kole et al., 2022; Piazzon et al., 2014; Wise et al., 2021). For turbot, two vaccines were developed using formalin-killed P. dicentrarch (Mabrok et al., 2023; Moustafa et al., 2010), and in one trial, the vaccine comprising antigen alone exhibited approximately 30% RPS. In another trial, when the vaccine containing Montanide ISA 763A adjuvant was combined with booster vaccination, the RPS increased to about 50%. In kelp grouper, immunization with the PLGA-encapsulated vaccine against U. marinum resulted in an impressive 80% RPS (Harikrishnan et al., 2012). Despite the high RPS values observed in the vaccines developed in the studies above, it remains uncertain whether these vaccines can effectively protect fish from infections caused by scuticociliates with multiple serotypes or bacterial co-infections frequently occurring in the field. To address this issue, we designed this trivalent vaccine targeting two serotypes of M. avidus and one co-infecting bacteria. We conducted a field test to evaluate its efficacy at four olive flounder farms on Jeju island. On farms B and D, where severe M. avidus and T. maritimum infections were experienced, a notable reduction in cumulative mortality rate was observed in the vaccinated groups. While the control groups exhibited cumulative mortality rates exceeding 65%, the vaccinated groups showed only approximately 30% mortality rate in both farms, demonstrating over 50% RPS values. On the other hand, farms A and C, which had relatively lower cumulative mortality rates below 20%, displayed even higher levels of protection in the vaccinated groups. The RPS values exceeded 70% in both farms, indicating a substantial reduction in mortality through vaccination during a moderate scuticociliatosis outbreak. To the best of our knowledge, this is the first time of field trial for the vaccine against scuticociliatosis. Our results underscore the potential of the developed vaccine as a valuable tool in managing and controlling M. avidus and T. maritimum infections in olive flounder farms. Furthermore, it is essential to assess the long-term effectiveness of the developed vaccine and investigate potential changes in pathogen strains and co-infection patterns over extended periods via continued monitoring and further research.
Conclusion
In conclusion, our study focused on the serotype distribution of M. avidus in the olive flounder farms on Jeju island, as determined through intensive disease monitoring between 2015 and 2020. The results revealed that M. avidus serotype I was predominant, accounting for 83% of cases, while M. avidus serotype II represented 17% of the observed serotypes. Additionally, we observed a significant co-infection rate of 58% with T. maritimum in fish affected by scuticociliatosis. Based on the monitoring data, we developed a trivalent vaccine against two serotypes of M. avidus and T. maritimum, which demonstrated remarkable efficacy with high RPS values under both laboratory conditions (ranging from 70% to 93%) and field trials (ranging from 52.64% to 76.38%). This vaccine could be a promising strategy for effectively managing and controlling M. avidus and T. maritimum infections, contributing to the overall health and sustainability of olive flounder farming.