Introduction
Valine (Val) is a branched-chain amino acid (BCAA), as well as an essential amino acid (EAA), with antioxidant and anti-inflammatory properties, which is utilized as a dietary supplement in aquaculture feed (Zhao et al., 2023). Val has been reported to play important roles in various physiological functions in animals including protein synthesis, energy production, immune functions, and tissue repair (Zhang et al., 2017). The effect of Val on animal immunity has been extensively studied; for example, Chen et al. (2017) found that Val is involved in macrophage phagocytosis and plays a prominent role in inhibiting arginase activity to stimulate nitric oxide production. Several studies have found that optimum dietary Val levels can enhance growth and feed utilization in fish and shrimp species, including, black tiger shrimp (Penaeus monodon) (Millamena et al., 1996), red seabream (Pagrus major) (Rahimnejad & Lee, 2013) and largemouth bass (Micropterus salmoides) (Zhao et al., 2023).
Val is one of the most limiting EAAs in black tiger shrimp (Millamena et al., 1996) since it cannot be biologically synthesized in vivo; therefore, dietary supplementation of sufficient amounts of Val is required in aquafeeds (Coloso & Cruz, 1980; NRC, 2011). In fish, dietary Val deficiency has resulted in limited growth and poor non-specific immune responses in rainbow trout (Oncorhynchus mykiss) (Ahmad et al., 2021), hybrid grouper (Epinephelus fuscoguttatus ♀ × Epinephelus lanceolatus ♂) (Zhou et al., 2021) and Nile tilapia (Oreochromis niloticus) (Xiao et al., 2017). An excess of dietary Val was reported to affect negatively on the growth performance and innate immunity in golden pompano (Trachinotus ovatus) (Huang et al., 2017). Even though, the effects of dietary Val on fish immunity are well understood (Luo et al., 2014; Xiao et al., 2017), the use and efficacy of Val supplementation to enhance shrimp immunity are poorly described.
Fish meal (FM) has been considered as the supreme protein source in aquaculture industry owing to its excellent quality of protein, such as high crude protein and well-balanced amino acid (AA) profile. However, it has become unsustainable to utilize FM as a main feed protein source in aquafeeds mainly due to higher price and increased demand of pelagic fishes for human consumption (Olsen & Hasan, 2012). One potential mode of utilizing plant protein sources replacing FM is to supplement or fortify the diets with EAA. Understanding and quantifying the EAA requirements of shrimp is critical when optimizing least cost feed formulations in aquaculture industry.
Pacific white shrimp (Penaeus vannamei) is one of the most important shrimp species in global aquaculture. The requirements of EAAs such as arginine (Zhou et al., 2012), lysine (Xie et al., 2012) and threonine (Zhou et al., 2013) for P. vannamei have already been investigated. Nonetheless, to the best of our knowledge, the dietary Val requirement has not been determined for P. vannamei. Therefore, this study aimed to determine the optimal dietary Val requirement based on the growth performance, feed utilization efficiency, protein synthesis and non-specific immune responses of juvenile P. vannamei.
Materials and Methods
The formulation and nutrient composition of the experimental diets (Table 1) were based on the protein requirements of P. vannamei (Lee & Lee, 2018). A basal diet was formulated using a Val-free crystalline AA mixture and 5% squid liver powder was added to enhance the palatability of the semi-purified diets. Considering the Val contributions from possible feed ingredients, crystalline Val was incorporated to the basal diet with graded levels of 0, 4, 8, 12, and 16 g/kg diet at the expense of L-glycine, to achieve targeted Val levels of 3, 6, 9, 12, and 15 g/kg diet, respectively. The analyzed actual Val concentrations in the experimental diets were 2.7, 5.1, 8.7, 12.1, and 16.0 g/kg of diet, respectively. All dry ingredients were mixed well and the fish oil was added with a feed mixer (NVM-14, Daeyung, Seoul, Korea), followed by distilled water (7%). The mixed wet dough was extruded to a size of 2 mm using a pelleting machine (SP-50, Kumkang Engineering, Daegu, Korea), dried at 24°C for 7 h and then stored at –24°C until use.
Free amino acid mixture (g/kg): contains 66.8 g arginine, 26.7 g histidine, 44.9 g isoleucine, 83 g leucine, 108.6 g lysine, 29.5 g methionine, 44.4 g phenylalanine, 45.9 g threonine, 6.7 g tryptophan, 103.4 g aspartic acid, 151.5 g glutamic acid, 37.8 g serine, 32.6 g proline, 75 g glycine, 76.2 g alanine, 35.1 g tyrosine and 31.9 g starch.
Mineral premix (g/kg of mixture): ferrous sulphate, 10.0; copper sulphate, 1.0; zinc sulphate, 30; manganous sulphate, 2.0; cobalt chloride, 10.0; potassium iodide, 1.0; potassium, 6.0; sodium selenite, 0.01.
Juvenile P. vannamei were acclimated to experimental condition for one week. After that, healthy and similar sized shrimp (average body weight, 0.46 g) were randomly distributed into 20 tanks (120 L capacity) with 12 shrimp per tank. Quadruplicate groups of shrimp were fed the diets 4 times daily (08:00, 11:30, 15:00, 18:30 h) at 2%–5% of total body weight for 8 weeks. The total weight and total number of shrimp in each tank were measured every three weeks to adjust feed amounts per experimental tank based on specific growth rate (SGR). Shrimp were fasted for 18 h to prior the measurement of their weight or sampling to minimize stress. The photoperiod was maintained at 12:12 (light:dark), and continuous aeration was supplied to each tank to maintain enough dissolved oxygen (DO) levels. During the feeding trial, rearing water quality was maintained by exchanging 70%–80% of rearing water every three days with pre-heated sea water except for the first 6 days. The water quality was maintained as follows; temperature 29.6°C–30.6°C, DO 5.56–6.01 mg/L, salinity 33 ppt and pH 7.65–7.81. Total ammonia nitrogen was kept below 0.08 mg/L during the feeding trial by changing the rearing water.
After 8 weeks of the feeding trial, all the shrimp in each tank were weighed individually to calculate final body weight (FBW), weight gain (WG), SGR, protein efficiency ratio (PER), feed conversion ratio (FCR) and survival. Eight shrimp from each tank were randomly captured and anesthetized by dipping in ice water for 3 min. Hepatopancreas were collected from four shrimp under sterile conditions, frozen immediately in liquid nitrogen and stored at –84°C for analysis of relative gene expression: prophenoloxidase (proPO), lysozyme, crustin and insulin-like growth factor binding protein (IGF-BP). Four shrimps were sampled additionally and immediately frozen (–84°C) for the analysis of whole-body proximate compositions and AA concentrations.
Analysis of dry matter, ash and crude lipid were performed according to the Association of Official Analytical Collaboration (AOAC) (2005). Crude protein was quantified by using an automatic Kjeltec Analyzer Unit 2300 (Kjeltec 2300, FOSS analytical, Hillerød, Denmark). AA compositions in the diets and whole-body samples were determined using an automatic AA analyzer (S433, Sykam GmbH, Fürstenfeldbruck, Germany).
Total RNA isolation, cDNA synthesis, and gene expression of hepatopancreas tissue were conducted following the methods described by Hasanthi & Lee (2023). Primer sequences for the analyzed genes are shown in Table 2, and β-actin was used as the reference gene. Relative mRNA expression levels were calculated using the 2-ΔΔCT method. Analysis of gene expression was conducted using qRT-PCR (TaKaRa, Shiga, Japan).
The data were subjected to one-way analysis of variance (ANOVA) using SPSS version 24.0 (SPSS, Chicago, IL, USA). Data of each parameter were initially checked for normality. Mean differences among dietary groups were determined by Duncan’s multiple range test, with significance at p < 0.05. A polynomial regression model and broken-line regression model were used for the determination of optimum dietary Val level based on shrimp FBW. The data were presented as mean ± SD. The percentage data were transformed into arcsine values prior analysis. Orthogonal polynomial contrast was used to assess whether the effect was linear and/or quadratic.
Results
Growth performance, feed efficiency and relative expression of shrimp IGF-BP are presented in Table 3. Results of the 8-week feeding trial showed that shrimp growth performance was increased when increasing dietary Val level up to 12.1 g/kg diet, whereas the lowest FBW, WG, and SGR values were observed in shrimp fed 16.0 g/kg Val diet; however, did not significantly differ from the 2.7 and 5.1 g/kg Val groups. PER was significantly increased in shrimp fed 8.7 and 12.1 g/kg Val diets than in other groups. Shrimp fed 8.7 and 12.1 g/kg Val diets exhibited significantly lower FCR than the 2.7 and 16.0 g/kg Val group. There was no significant difference in daily feed intake (DFI) across all groups. IGF-BP relative gene expression of 8.7 g/kg Val group was significantly enhanced compared to that of shrimp fed all other diets. Shrimp survival varied from 73% to 77% and was not significantly affected by Val supplementation. The FBW, WG, SGR and relative expression of IGF-BP showed significant quadratic trends with Val levels.
Whole-body protein level was significantly influenced by Val levels, whereas moisture, crude lipid and ash levels were not significantly affected (Table 4). Protein level was significantly lower in 2.7 g/kg Val group than in all other Val groups. Whole-body protein levels displayed significant quadratic and linear trends when increasing dietary Val levels. Whole-body Val, phenylalanine and threonine concentrations were significantly affected by dietary Val levels (Table 5). Shrimp fed 2.7 g/kg Val diet exhibited significantly lower whole-body Val concentration than other groups.
The effects of different levels of dietary Val on immune related gene expression are shown in Fig. 1. A significantly increased expression of proPO gene was observed in shrimp fed 8.7 and 12.1 g/kg Val diets than shrimp fed 2.7 g/kg Val diet. Lysozyme gene expression was significantly higher in shrimp fed 5.1 and 8.7 g/kg Val diets than shrimp fed 2.7 g/kg Val diet. Crustin gene expression was significantly increased in shrimp fed 8.7 g/kg Val diet compared to that in shrimp fed 2.7 g/kg Val diet. Overall, the gene expression levels of proPO, lysozyme and crustin were lower in shrimp fed the lowest Val level, 2.7 g/kg Val, than shrimp fed diets containing higher Val levels.
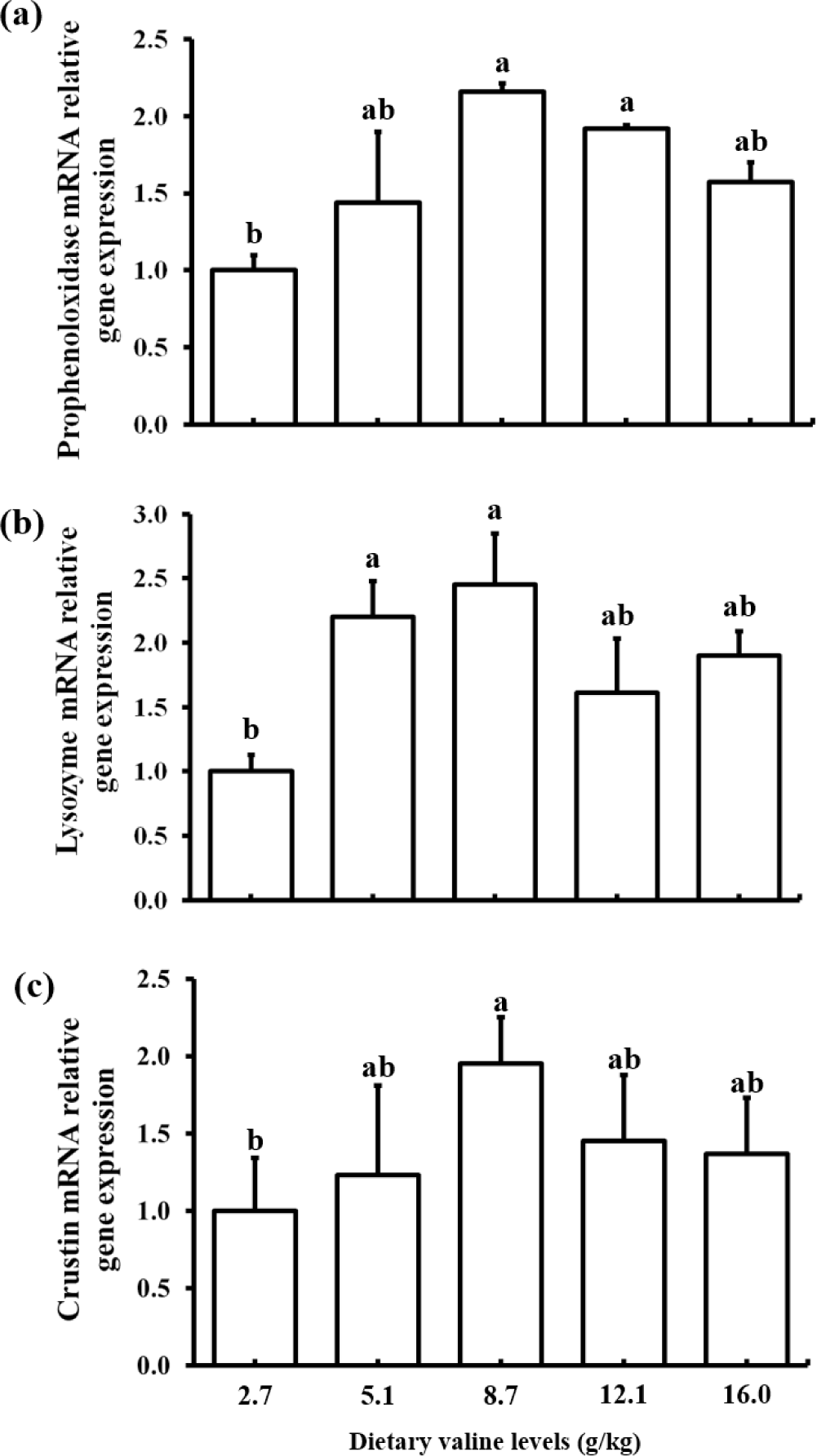
The polynomial regression results showed that the dietary Val requirement of juvenile P. vannamei (1.32–1.55 g) was 9.54 g/kg diet for the maximum FBW or 27.2 g/kg dietary crude protein level (Fig. 2). Broken-line regression analysis showed that the maximum FBW occurred at 9.27 g/kg of Val (26.2 g/kg based on protein level).
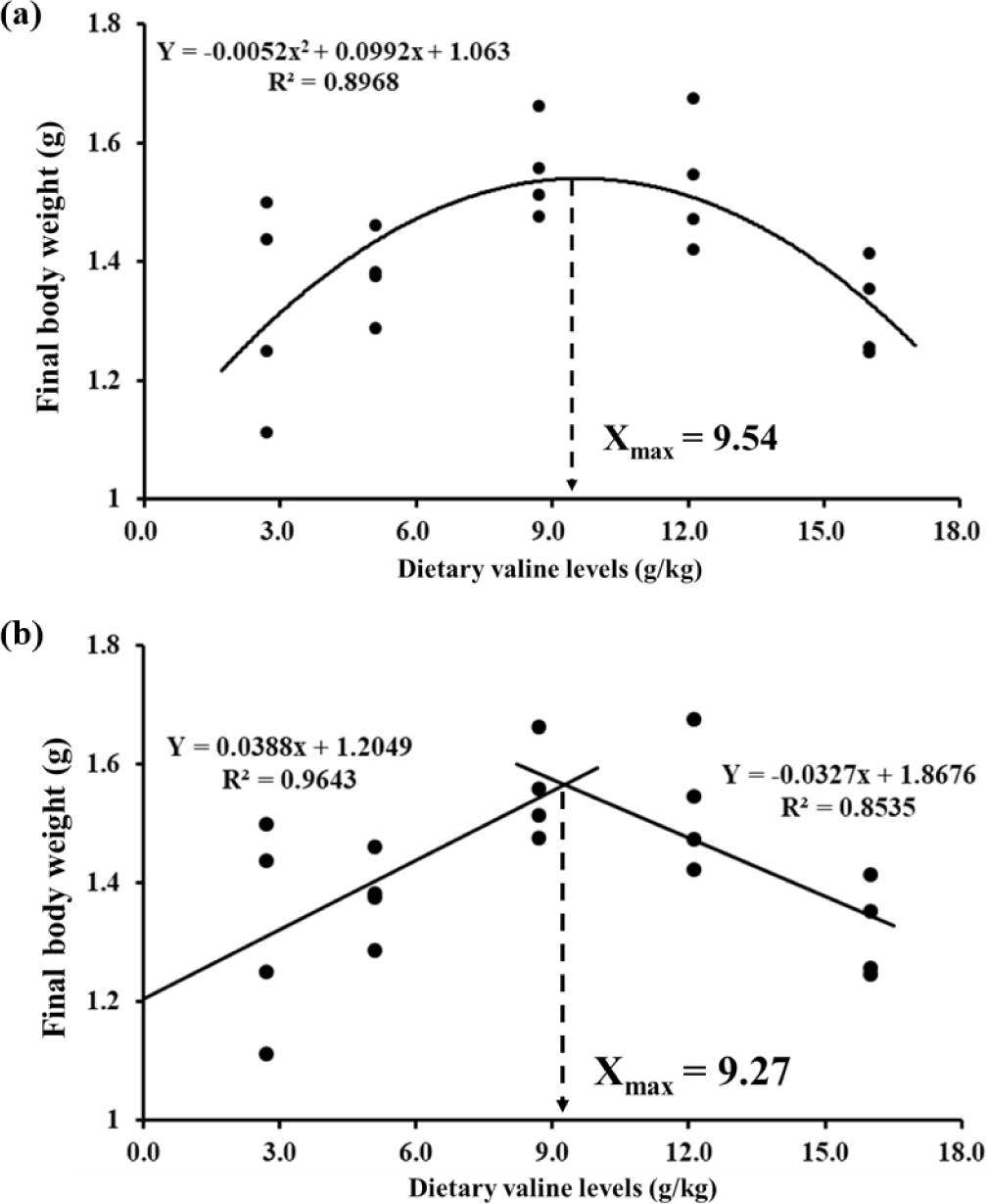
Discussion
In this study, a decrease in growth performance and feed utilization efficiency was observed in shrimp fed Val-deficient diets which was likely to be lower than 5.1 g/kg, whereas the performance was increased with dietary Val level up to 12.1 g/kg diet. Similar results were reported in other shrimp species and fishes, such as black tiger shrimp (Millamena et al., 1996), golden pompano (Huang et al., 2017) and hybrid grouper (Zhou et al., 2021). The estimated Val requirement level (9.27–9.54 g/kg) in this study was similar to those previously reported for rainbow trout (8–16 g/kg) (Rodehutscord et al., 1997), lake trout (Salvelinus namaycush) (8–10 g/kg) (Hughes et al., 1983) and channel catfish (Ictalurus punctatus) (7.1 g/kg) (Wilson et al., 1980). However, this value is lower than those reported for black tiger shrimp (13.5 g/kg) (Millamena et al., 1996) and several other fish species, such as grass carp (Ctenopharyngodon idella) (14.0–14.7 g/kg) (Luo et al., 2014), golden pompano (19.9–20.1 g/kg) (Huang et al., 2017) and hybrid grouper (15.6 g/kg) (Zhou et al., 2021). Abidi & Khan (2004) reported that Val requirement varies with growth stage and differences in species. The Val requirement for 1.57–5.68 g and 498 g rainbow trout were 18.2 g/kg and 14.1–15.0 g/kg, respectively (Ahmad et al., 2021; Bae et al., 2012). Therefore, it is necessary to evaluate Val requirements according to various factors.
IGF-BP is a family of proteins that bind to insulin-like growth factors (IGFs) to modulate their bioavailability and activity; they are produced primarily in the liver (Holly & Perks, 2006). In the present study, dietary Val supplementation was found to upregulate the expression of IGF-BP genes in shrimp. This upregulation might have occurred because Val activates the mammalian target of rapamycin (mTOR) pathway. mTOR is a major signaling pathway that regulates cell growth, proliferation, and metabolism (Zou et al., 2020). Val was reported to activate mTOR pathway through the regulation of phosphorylation of eIF4E-binding proteins and S6K1 in bovine mammary epithelial cells (Zhou et al., 2018). Zhou et al. (2021) also found that dietary Val supplementation upregulated the growth hormone production and receptor expression, IGF-1 secretion and the expression of TOR-related signaling molecules. In this study, shrimp group fed 9 g/kg Val diet showed the highest relative mRNA expression of IGF-BP genes along with the highest growth performance. Val supplementation may contribute to the growth-promoting effects in shrimp by upregulating IGF-BP gene expression.
In diets, a deficient or excess level of EAAs, i.e., Val, has shown to negatively affect shrimp and fish growth performance (Ahmad et al., 2021; Millamena et al., 1996). For example, previous studies demonstrated that EAA deficiencies can led to decreased growth performance in P. vannamei (Jin et al., 2017; Zhou et al., 2013). Dietary Val deficiency (10 g/kg dry matter basis) showed a significantly reduced growth performance in black tiger shrimp (Millamena et al., 1996) and limited the growth of fishes (Huang et al., 2017; Zhou et al., 2021). However, our results showed that an excess of dietary Val (> 12.1 g/kg) can also negatively affect the growth performance of P. vannamei, thus suggesting that moderate concentrations of dietary Val are desirable to avoid negative growth consequences. Val, leucine (Leu) and isoleucine (Ile) have similar side chain structures; therefore, an excess supplementation of each BCAAs can cause antagonistic effects as they compete with each other for common transport carriers and catabolic enzymes in the body (Matsumoto et al., 2014; Zhang et al., 2017). Han et al. (2014) found that an excess dietary Val or Leu level negatively affected the growth performance of olive flounder (Paralichthys olivaceus) as previously reported in chinook salmon (Oncorhynchus tshawytscha) (Chance et al., 1964). In the present study, antagonism among BCAAs may have contributed to the reduced growth of P. vannamei at higher dietary Val supplementation levels. In addition, over consumption of one single AA can lead to an extra energy consumption for its deamination and excretion (Ahmed & Khan, 2006). According to previous studies, excess arginine, tryptophan or Leu in diets decreased the growth performance of P. vannamei (Jin et al., 2017; Liu et al., 2014; Zhou et al., 2012). It was suggested that an excess level of dietary individual AA can interfere the absorption of other nutrients resulting in a higher catabolism or poorer utilization of protein in the body (Choo et al., 1991). Therefore, we recommend a further study to investigate individual AA digestibility including Val in shrimp.
A well balanced EAA composition facilitates the protein synthesis; Val can regulate skeletal muscle protein metabolism together with Leu and Ile in rats (Donato et al., 2006). Ahmed & Khan (2006) reported the importance of Val supplementation in diet for protein synthesis in Indian major carp (Cirrhinus mrigala). PER is a major determinant of body WG in shrimp (NRC, 2011). In our results, optimal dietary Val levels improved PER and whole-body protein synthesis in shrimp, along with a similar trend in WG. These results are consistent with those reported for Nile tilapia (Xiao et al., 2017), red sea bream (Rahimnejad & Lee, 2013) and hybrid grouper (Zhou et al., 2021). However, excessive dietary Val decreased whole-body protein synthesis and PER indicating that an excess level of dietary Val could impair normal protein metabolism rather than improving the protein synthesis (Xiao et al., 2017) as reported in blunt snout bream (Megalobrama amblycephala) (Ren et al., 2015).
In the present study, an imbalance (deficiency or excess) of dietary Val had a negative effect on innate immunity (proPO, crustin and lysozyme) of shrimp. AAs play important roles in metabolic pathways involved in the innate immune response, as reported in shrimp (Jin et al., 2017; Liu et al., 2014). The proPO activation system is known to be a major component of the invertebrate immune system (Amparyup et al., 2013). The proPO gene expression in hepatopancreas was upregulated significantly with increasing dietary Val levels of up to 8.7 g/kg, but decreased at 16.0 g/kg in the present study. This finding suggests that optimal levels of dietary Val could have a positive effect on proPO activity in P. vannamei. Similar result was reported in P. vannamei fed diets varying threonine levels (Zhou et al., 2013). Shrimp have developed innate immunity, which directly improves disease resistance (Amparyup et al., 2013). Lysozyme and crustin are two important peptides in the shrimp antimicrobial defense system and play a major role in combating invading pathogens and preventing diseases (de Lorgeril et al., 2008). Anti-microbial peptides are components of the innate immune system that protect organism against a variety of pathogens, including gram-positive bacteria and fungi (Tassanakajon et al., 2011). In the present study, lysozyme and crustin expression significantly increased with increasing dietary Val concentrations up to 8.7 g/kg; however, a numerically decreased expression was observed with higher level of dietary Val up to 16.0 g/kg. Thus, an imbalance (deficiency or excess) in dietary Val could adversely affect the innate immunity of shrimp, as reported in golden pompano (Huang et al., 2017) which were fed a Val deficient diet (15.0 g/kg dry matter basis).
Millamena et al. (1996) reported that Val had a major effect on the survival of black tiger shrimp. In this study, the survival of shrimp increased with increasing dietary Val concentration, but was not significant. This could be attributed to the various functions of Val on the immune response, Val is predominantly involved in generating propionyl-CoA which is a glycogenic precursor of succinyl-CoA (Ahmed & Khan, 2006). A previous study found that Val supplementation increased macrophage phagocytosis through the activation of PI3K/Akt1 and nitric oxide production in mice (Chen et al., 2017). In infected mice, optimal Val supplementation resulted in an enhanced macrophage phagocytosis on several bacterial pathogens including Pseudomonas aeruginosa (Chen et al., 2017). In addition, mice fed Val-deficient diets showed an increased susceptibility to bacterial infection demonstrating the anti-microbial activity of Val in a certain circumstance (Petro & Bhattacharjee, 1981). Val is involved in the production of biochemical compounds in the body that primarily aid in energy production (Ahmed & Khan, 2006). Studies on the effects of dietary Val on shrimp immune function are scarce. In this study, we showed how different levels of dietary Val could affect several innate immune responses in shrimp to address these research gaps, and obtained a promising result that could be a preliminary data for further studies on individual AA requirement of the shrimp.
In conclusion, an optimal level of dietary Val positively affected growth performance, feed utilization efficiency and the immune response of P. vannamei. Specifically, the optimal Val requirement level was estimated to be 9.27–9.54 g/kg (dry matter basis, 26.2–27.2 g/kg dietary protein) by polynomial regression and broken-line regression analyses of FBW.