Introduction
The striped catfish (Pangasius hypophthalmus) is considered a superior aquaculture species for tropical regions throughout Southern Asia. Although this species originated in Vietnam, it has been successfully cultured in other tropical countries, including Indonesia, due to its adaptability to various environmental conditions (Zannat et al., 2023). It also offers promising prospects in both domestic and international markets as skinless and boneless fillets. The primary markets for this commodity are the EU, the USA, Brazil, Mexico, China, and Hong Kong (Platas-Rosado et al., 2021). To increase the productivity and sustainability of aquaculture practices associated with this fish, it will be crucial to optimize the growth performance and feed efficiency through feeding strategies. One of the challenges encountered in fish farming is the high cost of feed, which represents a significant proportion of the total production cost. In fact, the total cost of feed can reach up to 60–70% of the overall production costs. Therefore, developing an alternative strategy to improve the feed efficiency and growth performance of striped catfish while minimizing production costs is necessary.
Kombucha is a fermented tea widely studied to contain various types of microorganisms, offering its potential as a probiotic. Lactic acid bacteria (LAB), such as Lactobacillus sp. and Lactococcus sp., that are found in kombucha (Bogdan et al., 2018), which are commonly used as probiotic bacteria in aquaculture (Leong et al., 2023). Moreover, kombucha contains various beneficial compound, such as organic acids, vitamins, and minerals, that contribute to fish health. To date, the use of kombucha as a probiotic in aquaculture has not been extensively studied. Aprianus et al. (2021) and Ramadhan et al. (2021) reported that kombucha can be used to improve growth performance and protein and fat retention of Clarias sp. Dietary kombucha is expected to increase fish digestibility with the help of exogenous enzymes derived from various microorganisms in kombucha. This increased digestibility is associated with increased protein and fat retention in the fish. Overall, higher protein and fat retention indicates more protein and fat in feed are absorbed and stored in the form of meat.
Previous study has indicated that kombucha can positively influence the growth parameter and feed efficiency (Aprianus et al., 2021; Ramadhan et al., 2021). This study aims to compare the effectiveness of kombucha supplementation in feed with different protein content on the growth performance and feed efficiency of striped catfish. By investigating the interaction between kombucha supplementation and dietary protein content, this study sought to provide insight into optimizing feed formulations for better growth outcomes in striped catfish aquaculture and minimizing feed costs.
Materials and Methods
This study was conducted in March-July 2023 at the Labo-ratory of the Faculty of Health, Medicine, and Life Sciences, Airlangga University in Banyuwangi. This research has received ethical approval and oversight from Universitas Airlangga Faculty of Dental Medicine Health Research Ethical Clearance Commission under authorization number 1089/HRECC.FODM/IX/2023.
A total of 400 juvenile striped catfish (P. hypophthalmus; length 9.97 ± 0.20 cm and weight 9.09 ± 0.44 g) obtained from local fish farmer Glenmore, Banyuwangi, Indonesia was randomly distributed into 20 tanks and acclimatized for 7 days. During the acclimation period, a commercial pellet containing 28% protein (Haida, PT. Haida Agriculture, Pasuruan, Indonesia) was provided two times a day (08.00 a.m and 16.00 p.m) until satiation. The tank was aerated and maintained at optimum water quality parameters for fish growth as follows: water temperature at 29–31°C, dissolved oxygen level > 4 mg/L, ammonia < 0.5 mg/L, and pH within 7.01–8.05.
Two kinds of commercial pellets with different protein levels were used as the experimental diet. The low-protein pellet contained ± 28% protein (Haida, PT. Haida Agriculture) and the high-protein pellet contained ± 39% protein (Prima Feed, PT. Matahari Sakti, Surabaya, Indonesia). A total 20 mL of kombucha tea, was obtained from Ibu Bumi, Banyuwangi, Indonesia, mixed with 0.2% binder (Progol, Indosco, Sidoarjo, Indonesia). Both kombucha and binder were sprayed evenly onto the one kg of both commercial pellets (28% and 39% protein). While, diet treatment without kombucha supplementation were prepared by spraying 20 mL of distilled water and binder onto the commercial pellets. Pellets were dried at room temperature for 30 min and were stored in a refrigerator at 5°C before being used in the experiment. The proximate composition of the experimental diet was analysed using the Association of Official Analytical Chemists (AOAC) standard method (AOAC, 2005). Crude protein was determined by the Kjeldahl method, crude lipid by ether-extraction using Soxhlet apparatus, dry matter content was measured by drying at 105°C for 24 h, ash content by burning the specimens at 550°C for 5 h, and crude fiber was measured by calcining undigested specimens from sulfuric acid and sodium hydroxide solution. The proximate composition of the experimental diet was presented in Table 1.
Prior to the experiment, fish were fasted for 24 h. 20 tanks of fish were divided into 4 groups, i.e., PaKa was fed with low protein (± 28%) without kombucha addition, PaKb was fed with low protein (± 28%) enriched kombucha, PbKa was fed with high protein (± 39%) without kombucha addition, and PbKb was fed with high protein (± 39%) enriched kombucha. Each treatment has five replications. Fish were maintained for 40 days and fed with experimental diets three times a day at satiation (Fatmawaty et al., 2021). Faecal removal was siphoned every day to maintain the optimum rearing water quality. The water parameters, including temperature, pH, dissolved oxygen, and ammonia, were monitored daily and maintained at 29–31°C, dissolved oxygen level > 4 mg/L, ammonia < 0.5 mg/L, and pH within 7.01–8.05.
The body weight of fish was measured at intervals of 10 days during the rearing period. All fish were starved for 24 h prior to weight measurement. The parameters observed for growth assessment are weight gain (WG), specific growth rate (SGR), total feed consumption (TFC), and feed conversion ratio (FCR). TFC was noted every day and calculated in the end of the rearing period. Growth parameters are calculated using the formula as described by Ramadhan et al. (2021):
where Wt = final weight (g); Wo = initial weight (g); and t = length of rearing period (days).
At the end of the experiment, five fish were randomly taken from each of five replication tanks per treatment group (n = 25) and the liver was removed and weighed for calculating hepatosomatic index (HSI) according to Budi et al. (2015). The body composition of fish, including protein and lipid content, were analyzed using the recommended methods of the AOAC (AOAC, 2005). Crude protein was determined by the Kjeldahl method and crude lipid by ether-extraction using Soxhlet apparatus. The protein and lipid retention were calculated using the formula described on Aprianus et al. (2021).
At the end of the experiment, five fish from each treatment were randomly collected and anesthetized using ice slush before being dissected. An intestine sampling tissue for histological examination was carried out from anterior intestinal samples (1 cm after the stomach) and fixed in 10% neutral buffered formaldehyde solution. The intestinal tissue sample underwent various steps, including dehydration, embedding in paraffin wax, slicing using a microtome, and staining with Mayers Hematoxylin Eosin. Intestinal observation was performed using a microscope (Eclipse E200-LED, Nikon, Tokyo, Japan) with 400x magnification, including villi length (VL), villus apical and basal width, intestinal diameters (D), and number of villi (N). Villus surface area (VSA) was calculated as described by Iji et al. (2001).
A total intestinal bacteria analysis was performed to compare the total intestinal microbes in fish fed with kombucha to fish fed without kombucha. It was carried out using the method as described by Alishahi et al. (2018) with a slight modification. At the end of the experiment, 0.1 g of intestinal sample was homogenized in 9.9 mL of sterile saline. Serial dilutions of intestinal content were prepared from 10–2 to 10–7 in sterile saline. A total 0.1 mL of each dilution were spread evenly on triptic soy agar (TSA) medium. Then, TSA plates were incubated at 35°C for 24 h. The colonies of bacteria growing on TSA media were counted using a hand-counter and calculated as colony forming units (CFU/mL).
Data, including growth performance, feed efficiency, HSI, intestinal microbes, and intestinal histomorphology, were analyzed using SPSS software (SPSS version 20 for Windows, Chicago, IL, USA) by two-way ANOVA. Significance differences was noted at p < 0.05. The results are presented as mean value with standard deviation (SD). Proximate composition of experimental diets was analyzed descriptively.
Results
The growth performance and FCR of striped catfish after 40 days of feeding trial are presented in Table 2. The WG and SGR have a similar trend. The PbKb group has the highest WG (15.33 ± 1.20 g) and SGR (2.46 ± 0.15%), significantly higher than the other groups. The lowest WG and SGR were observed in the PaKa group at 7.94 ± 0.44 g and 1.59 ± 0.08%, respectively. The two-way ANOVA indicated that protein level and kombucha supplementation significantly affected WG and SGR, while the interaction was insignificant. This proved that increased protein levels in diet and kombucha supplementation independently contribute to increased WG and SGR. The TFC varied among the groups but did not show as strong a trend as WG and SGR. The highest TFC was in the PbKb group (17.89 ± 0.89 g) and the lowest was in the PaKa group (15.64 ± 0.33 g). Meanwhile, the best FCR was in the PbKb group at 1.18 ± 0.14. The best FCR is characterized by a lower FCR value, which indicates that fish used feed most efficiently to gain weight.
Two-way ANOVA | |||||||
---|---|---|---|---|---|---|---|
Protein level (P) | p < 0.00 | p < 0.00 | p < 0.08 | p < 0.00 | |||
Kombucha (K) | p < 0.00 | p < 0.00 | p < 0.12 | p < 0.00 | |||
P × K (Interaction) | p < 0.24 | p < 0.15 | p < 0.64 | p < 0.01 |
The HSI of striped catfish after 40 days of feeding treatment is presented in the Fig. 1. The PbKb group showed the highest HSI at 2.61 ± 0.03% and was significantly different to other treatments. The addition of kombucha to the both-protein diet resulted in a notable increase in the HSI. The increasing protein diet treatment also showed increasing HSI. The result indicates that higher protein diets and the inclusion of kombucha independently and synergistically increase the HSI (p < 0.05), suggesting improved liver function.
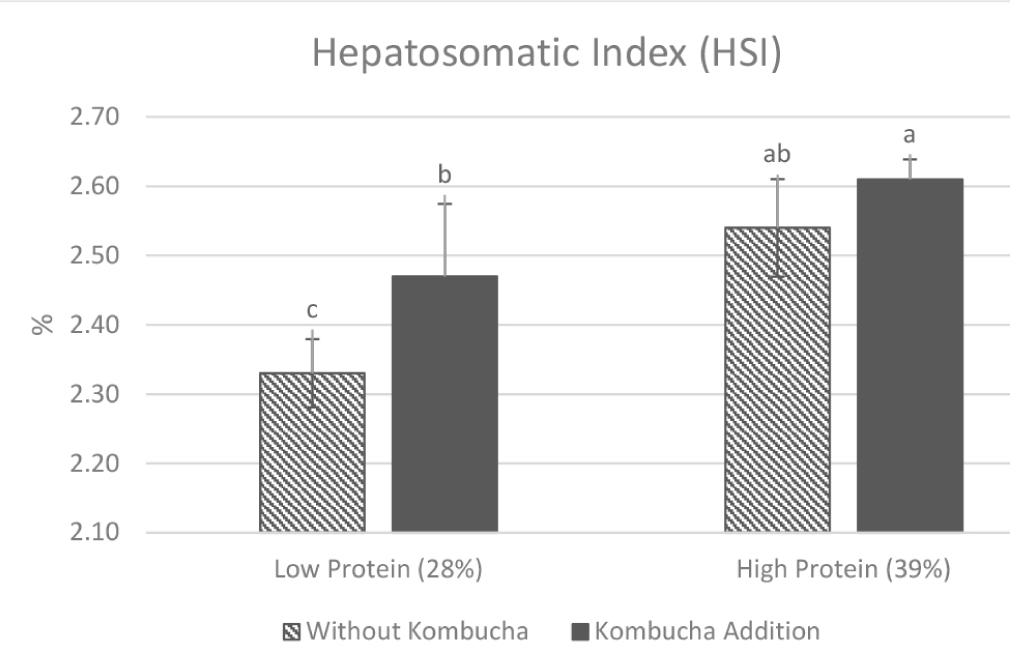
The body composition of catfish, including protein and lipid retention, after 40 days of feeding with an experimental diet is presented in Table 3. Protein content and protein retention (PR) in catfish varied significantly compared to other treatment. PbKb group generated the highest protein content and PR at 90.37 ± 7.12 g and 52.43 ± 5.73%, respectively. However, the lowest protein content and retention were observed in the PaKa group at 39.65 ± 1.20 g and 26.12 ± 1.60%. Lipid content and retention followed a similar trend as protein, with the highest lipid content and retention observed in the PbKb group while the lowest are in the PaKa group. The statistical analysis revealed that both protein level (p < 0.00) and kombucha supplementation (p < 0.00) independently gave significant effects on protein and lipid content and also the retention. However, the interaction between protein level and kombucha supplementation showed different effects. The interaction between protein level and kombucha supplementation was significant for protein content (p < 0.00), lipid content (p < 0.00), and lipid retention (p < 0.00), but not for PR (p < 0.58). These results indicate that higher protein diets and kombucha supplementation independently and significantly enhance protein and lipid accumulation, but their combined effect is particularly potent, especially with the PbKb treatment.
Two-way ANOVA | |||||
---|---|---|---|---|---|
Protein level (P) | p < 0.00 | p < 0.00 | p < 0.00 | p < 0.00 | |
Kombucha (K) | p < 0.00 | p < 0.00 | p < 0.00 | p < 0.00 | |
P × K (Interaction) | p < 0.00 | p < 0.58 | p < 0.00 | p < 0.00 |
The number of intestinal microbes after 40 days of treatment showed a positive impact of kombucha addition on both diet type (Fig. 2). In the low protein diet group, kombucha addition (PaKb) significantly enhanced the total microbial gut compared to without kombucha addition treatment (PaKa). The total microbes increased from 1.48 ± 0.07 × 106 CFU/mL to 1.82 ± 0.09 × 106 CFU/mL in the low protein group. Kombucha addition in high protein diet (PbKb) also led to a significant increase in microbial count (1.98 ± 0.08 × 106 CFU/mL to 2.09 ± 0.08 × 106 CFU/mL) than without kombucha treatment (PbKa), though the improvement was smaller than in the low protein group. PbKb group generated the highest microbial count among other treatment.
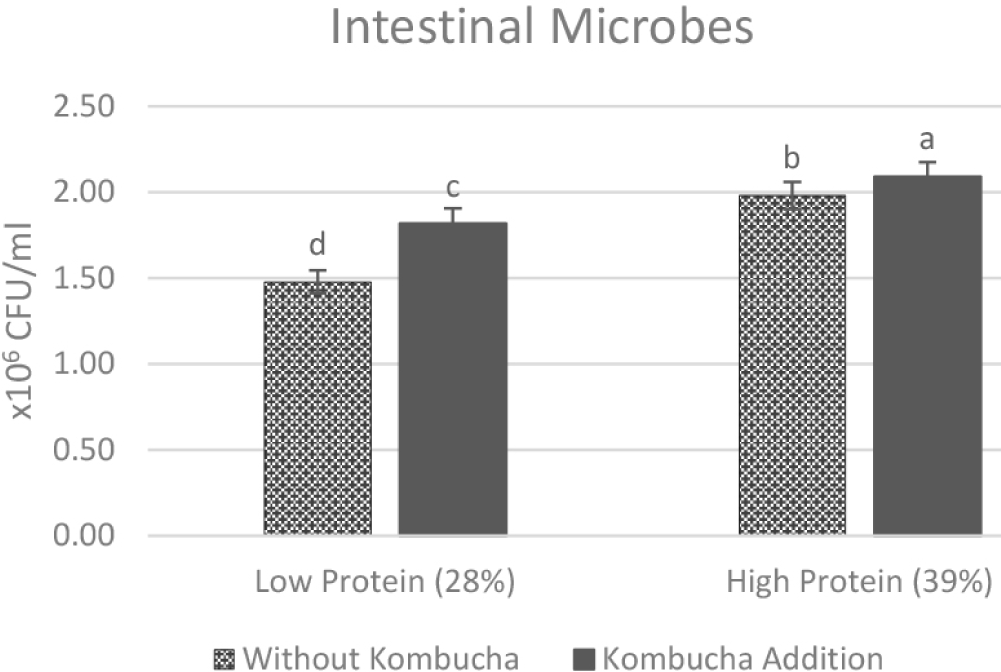
The intestinal histomorphology of catfish after 40 days of dietary treatment is depicted in Fig. 3. The histomorphology parameters observed were evaluated by examining several parameters, including VL, villus apical width (VAW), villus basal width (VBW), VSA, D and N as presented in Table 4. Overall, PbKb group exhibited the longest villi (55.15 ± 1.09 µm), the largest VSA (964.25 ± 78.98 µm2), intestinal diameter (222.04 ± 11.09 µm), and the highest N (25.40 ± 4.39). It was significantly different from other treatments. Both factors, protein level (p < 0.05) and kombucha supplementation (p < 0.05), independently increase VL, VSA, D and N. However, the interaction of both factors was synergic only at several parameters, including VL and D.
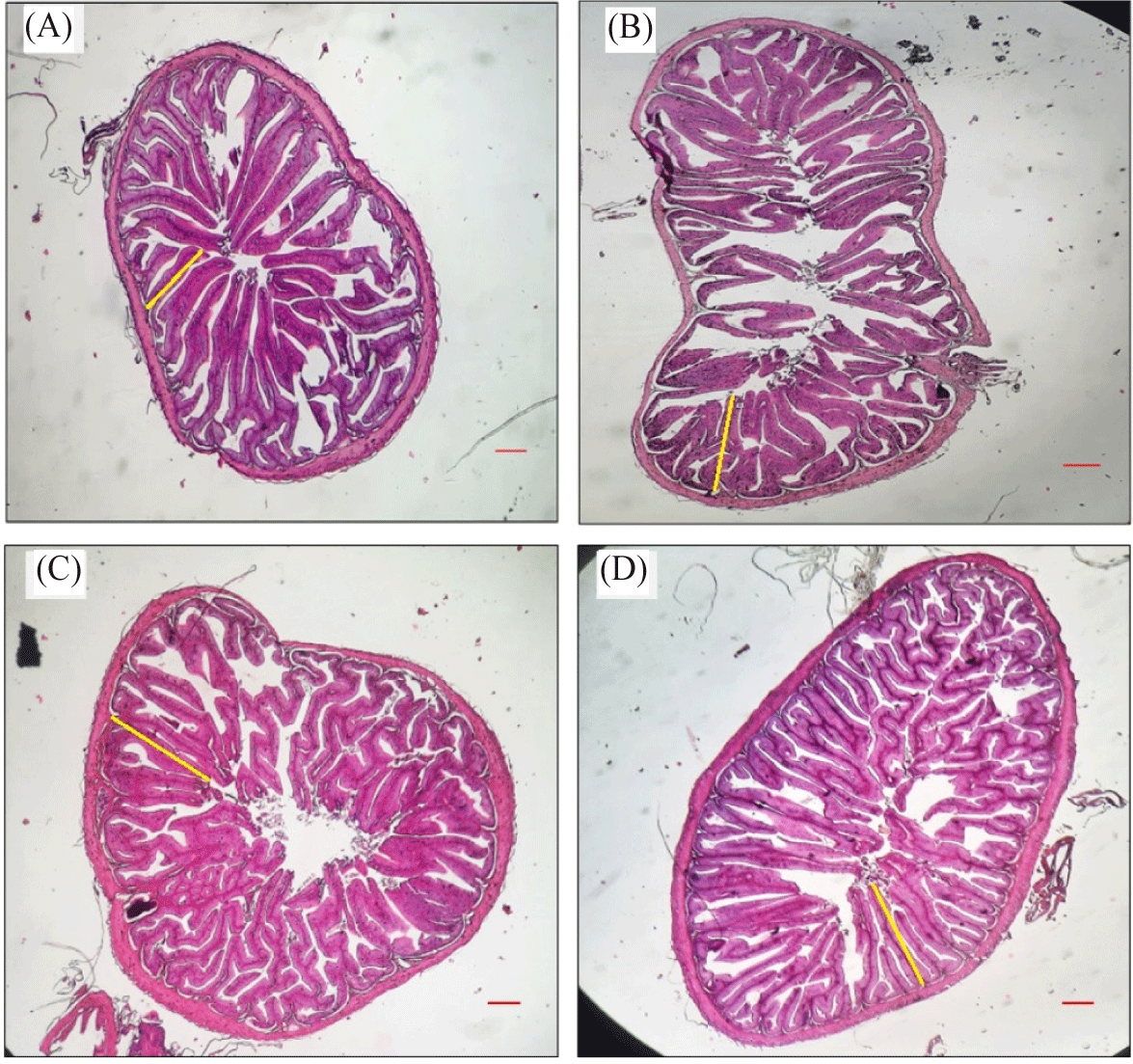
The feed efficiency and cost-effectiveness of each treatment were evaluated based on FCR, total feed required and total feed cost per ton of striped catfish production as presented in Table 5. Among the treatment, PaKb demonstrated the highest cost efficiency, with the total feed cost per ton of fish production at Indonesian Rupiah (IDR) 20.160.000. Conversely, PbKa had the highest total feed cost per ton at IDR 29.480.000, despite its relatively low FCR of 1.34, due to a higher feed price per kilogram. These result also proved that the kombucha addition on both diet appears to reduce feed cost per ton of fish production compared to their respective controls (PaKa and PbKa). PaKb has the lowest total feed cost with a 21.7% reduction compared to PaKa. Then, PbKb also shows a cost reduction, which is 6.4% lower than PbKa group.
Discussion
Kombucha supplementation and increasing protein levels in the diet significantly enhance the growth performance (WG and SGR) and feed efficiency (FCR) of striped catfish. A high-protein diet supplemented with kombucha results in the best performance. On the other hand, kombucha supplementation also improves WG, SGR, and FCR in low-protein diet treatments, leading to performance that is not significantly different from high-protein diet treatments. The improvement in growth performance is due to the increased feed conversion efficiency, indicated by the lower FCR values. This is influenced by several factors, including the high quality of feed, an optimal digestive system in the fish, and the performance of metabolic-related organs that regulate growth and energy storage (Yuru et al., 2020).
The quality of feed is not solely determined by its protein content but can also be enhanced through feed supplementation, such as the addition of kombucha in this study. This study also proved that kombucha supplementation enhances nutrient availability and digestive efficiency in both high and low protein diets. The growth improvement in the presence of kombucha in diet may be due to the probiotic properties of kombucha, which include beneficial microorganisms, especially LAB (Chakravorty et al., 2016; Puspawati & Arihantana, 2016; Yang et al., 2022). LAB has been recognized as a safe microorganism for humans and animals by FDA and European Food Safety Authority (EFSA). It has been applied in numerous aquatic host species and exhibited the ability to improve growth performance, immune system, and disease resistance (Chizhayeva et al., 2022). LAB also are known to produce exogenous enzymes such as protease, lipase, and amylase (Kieliszek et al., 2021). The protease generates the availability of amino acids in an intestinal environment, which can serve as the substrates for microbial metabolism and proliferation that affect fish gut microbiota (Wu et al., 2024).
In addition, the beneficial microbes in kombucha also interact synergistically with the existing gut microbiota and lead to a more diverse and abundant microbial community. Fish gut microbiota is a group of microorganisms normally found in the gastrointestinal tract that are closely associated with host’s growth and stress response. The composition of the gut microflora is influenced by the diet. This current study reported the supplementation of kombucha in both protein diet increased the number of intestinal microbes in fish (Fig 2). This finding is corroborated with previous study conducted by Zuenko et al. (2017) which reported the inclusion of external microbes (Bacillus subtilis) in fish feed increased total intestinal microbes by 13% in carp and 3.5 times higher in starlet (Acipenser ruthenus). Our study recorded the total intestinal microbes in striped catfish after kombucha inclusion reached 1.82 ± 0.09 × 106 CFU/mL in low protein diet and 2.09 ± 0.08 × 106 CFU/mL in high protein diet. This finding was higher than the study conducted by Haque et al. (2021) which reported the administered in-feed probiotic diet on the similar fish, P. hypophthalmus, only increased the total gut microbes at 9 × 105 CFU/mL. The increased intestinal microbial counts in our study demonstrated that the microbes in kombucha are able to pass through the fish digestive tract and colonize in the gut. The microbes will attach to the intestinal lining and produce several enzymes to break down the feed component into simple forms. The produced simple forms of nutrients not only benefit the fish but also provide a more nutrient-rich environment for gut microbes, so that it will promote microbial growth and proliferation (Ringø et al., 2022).
The highest number of intestinal microbes was recorded in the high protein diet supplemented with kombucha treatment (PbKb group). Meanwhile, the treatment without kombucha supplementation at both dietary protein content also displayed an improvement in the number of intestinal microbes as the dietary protein content increased. This confirmed that the number of gut microbes is not only influenced by kombucha supplementation but also by the protein level of the feed. Zhu et al. (2020) reported that different levels of protein diet changed the GIFT tilapia’s (Oreochromis niloticus) gut microbiome. In their study, a higher protein diet promoted intestinal microbial diversity compared to a low protein diet. This is due to higher protein intake can indeed stimulate the production of fish digestive enzyme. Studies on Pengba (Osteobrama belangeri) fry demonstrated that increased dietary protein levels up to 45% enhanced proteolytic enzyme activities (Ramesh et al., 2017). The improvement of digestive enzymes, especially proteolytic enzymes, generates the availability of amino acids in gut environment which can serve as the substrates for microbial metabolism and proliferation (do Vale Pereira et al., 2024).
An increase in microbial counts is not necessarily a positive outcome, as the overall impact depends on the composition of the microbial community. If harmful microbes, such as pathogenic bacteria, were to increase alongside beneficial microbes, the outcome could negatively affect fish health and growth. Pathogenic bacteria could cause diseases, impair nutrient absorption, or disrupt the gut microbiota balance, potentially leading to adverse effects on fish performance. In this study, the assumption that the increase in microbial counts was predominantly due to non-pathogenic, growth-promoting bacteria is supported by the absence of clinical signs of infection or mortality in the fish during the rearing period. This suggests that harmful microbes likely did not proliferate to a detrimental level. Additionally, the probiotic properties of kombucha, as demonstrated in other studies, may help mitigate the risk of harmful microbial growth by suppressing pathogens like Escherichia coli and Staphylococcus aureus through its antimicrobial compounds, including organic acids and phenolic compounds (Effendi et al., 2014; Hou et al., 2021). Furthermore, kombucha’s ability to modulate gut microbiota in favor of beneficial bacteria (e.g., Adlercreutzia) and enhance the production of short-chain fatty acids (SCFA; e.g., propionate) (Costa et al., 2022) underscores its potential role in maintaining gut health and supporting fish growth. Future studies could explicitly measure the relative abundance of pathogenic and beneficial microbes to confirm these interpretations and ensure that increases in microbial counts translate into positive outcomes.
A well-developed gut microbiota in fish contributes to shaping a better gut histomorphology, which plays an important role in nutrient absorption. This study proved that dietary kombucha supplementation not only increases the intestinal microbiota but also improves the morphology of the fish’s intestine. Histo-morphological analysis further showed that higher dietary protein level with kombucha supplementation treatment (PbKb) exhibited significantly longer villi, larger VSA, and higher intestinal diameter and N (Table 4). Some previous study reported that gut microbes affects intestinal morphology through several mechanisms, i.e inducing cell proliferation by producing bacterial metabolites, such as SCFA and vitamins, preventing intestinal villi damage by producing an antimicrobial compound and competitive exclusion of pathogenic bacteria, reduction intestinal inflammation by producing anti-inflammatory compounds, and enhancing protective barrier by stimulating mucus production by goblet cells (Diwan et al., 2023; Ringø et al., 2022).
In our study, higher protein level on diet (± 39%) was also significantly improved the fish intestinal histomorphology. Similar studies have been reported in tilapia (Oreochromis aureus; Michel-Parra et al., 2007) and silver catfish (Rhamdia quelen; Schneider et al., 2020), where diets with higher protein were shown to increase intestinal villus height. Intestinal morphology in fish can be influenced by feed quality, particularly amino acid composition and digestibility. Schneider et al. (2020) noted that a 4% reduction in dietary protein from 38% protein led to a decrease in villus height, despite this alteration was not statistically significant. Additionally, Cai et al. (2018) reported that increased villus height was associated with lysine intake from the diet. In our study, we suggest that the higher protein diet supplied more amino acids, including lysine, which may have contributed to enhanced intestinal development in the fish.
These improvements in intestinal morphology are indicative of enhanced nutrient absorption capabilities, as evidenced by the greater protein and lipid content of the fish as well as protein and lipid retention in this present study (Table 3). Simple nutrients, including amino acids, fatty acids, and sugars, are absorbed by villi and transported by blood to various tissues and organs for metabolic processes (Kuz’mina, 2021). The absorbed amino acids are used to build new proteins for growth and repairing tissue muscle. Fatty acids are stored as triglycerides in adipose tissue and have an important role as a component of cell membranes and can be used as an energy reserve. Meanwhile, sugars are used for immediate energy and the excess is stored as glycogen in the liver and muscle (Lall & Tibbetts, 2009). The body composition analysis revealed that the highest protein and lipid content were observed in the higher dietary protein level with kombucha supplementation treatment (PbKb). This result was similar to the protein and lipid retention of fish. Higher protein and lipid retention indicate the effectiveness of the protein and lipid conversion from the diet into fish body mass rather than being excreted as waste (Chen et al., 2023). The increased protein and lipid retention indicate that probiotics in kombucha facilitates better absorption and assimilation of these macronutrients. According to Aprianus et al. (2021), dietary kombucha in African catfish (Clarias sp.) also enhanced protein and lipid retention, which are related to the fish’s somatic growth. The enhancement of protein and lipid retention due to the administration of probiotics also reported in juvenile olive flounder (Paralichthys olivaceus; Niu et al., 2019), rainbow trout (Oncorhynchus mykiss; Sahraei et al., 2019), and Cirrhinus mrigala (Singh et al., 2022). In addition to kombucha, which has potential as a probiotic, protein and lipid retention can be influenced by other factors, including dietary composition. In our study, feed contained 39% of protein generates the best protein and lipid retention. This might be due to a high protein diet (39%) providing more essential and non-essential amino acids than a low protein diet (28%), which enhances protein accumulation in the fish body.
High dietary protein inclusion might also be responsible for increased lipid accumulation in the liver and whole body, as observed by HSI (Fig 1), lipid content and retention (Table 3). HSI is a measure of the relative size of the liver in comparison to the total weight of fish and is often used as an estimate of the energy status of the fish. According to Yuru et al. (2020), the liver is the main organ responsible for metabolizing and transforming nutrients, so its activity might have an impact on HSI. In our study, higher protein diets and the addition of kombucha increase the HSI, indicating improved liver function in metabolic activity and nutrient transformation. High protein diet lead to an increased amino acid catabolism and gluconeogenesis due to the converting excess amino acid into glucose for energy production or stored as glycogens (Melo et al., 2006). A higher HSI might represent a relatively larger liver with an indication that the liver stores excess glycogen and lipids which can be used to fulfil the energy requirements of the fish.
In this study, the increase in dietary protein is related to the increasing lipid content and lipid retention (Table 3). This was in line with the previous study reported on Rockfish (Sebastes schlegeli) by Li et al. (2023) and butter catfish (Ompok bimaculatus) by Chowdhury et al. (2022). Lipid retention in PbKb group reached more than 100%, suggesting that excess amino acid may be converted into lipid accumulation through lipogenesis. In fact, amino acids are classified as ketogenic, glucogenic and both ketogenic and glucogenic according to their metabolic fate. Ketogenic amino acids, such as lysine, are converted to acetyl CoA, a precursor to fatty acid synthesis. As reported by Teodósio et al. (2022), higher protein diets may provide more lysine, which is more easily catabolized than other amino acids that produce ketone bodies or acetyl-CoA. Furthermore, the addition of kombucha to the feed may increase the availability of lysine and other ketogenic amino acids, such as leucine, phenylalanine, tyrosine, tryptophan, and isoleucine. Studies by Jayabalan et al. (2010) and Jastrzębska et al. (2022) highlights that kombucha, beyond its probiotic microbial content, also contains active chemical compounds like free amino acids. This makes kombucha valuable not only as a probiotic source but also as a rich source of essential free amino acids. Dias et al. (2005) also reported that dietary protein sources affects the regulation of lipid metabolism in fish. Their study explained that European seabass (Dicentrarchus labrax)-fed fish meal-based diet has higher liver lipogenic enzyme activities, especially fatty acid synthase, which is responsible for the de novo synthesis of fatty acids.
Fish growth and tissue development are influenced by the protein and energy balance in the diet (Budi et al., 2015). The balanced energy can be achieved due to the presence of non-protein energy sources, mainly lipids and carbohydrates. An adequate lipid and carbohydrate level on diet ensures that fish use protein primarily for growth rather than energy, thereby improving PR. However, an optimal dietary protein level is more crucial for the best growth in practical aquaculture farming. According to Ahmed & Ahmad (2020), excessive protein in the diet can reduce the fish growth rate because excessive dietary protein may increase energy requirements for catabolism rather than for protein deposition. Moreover, protein is considered as the most expensive ingredient in fish feed, so that the surplus is considered wasteful, both biologically and economically.
Our study discovered the supplementation of kombucha facilitates better absorption in both low and high-protein diets due to its probiotic effect on enhancing intestinal microbiota and morphology. Moreover, it can reduce the feed cost as depicted in Table 5. The addition of kombucha resulted in a reduction in feed cost compared to the diet that did not include kombucha (PaKa and PbKa). Kombucha production can be relatively costly due to the raw materials and fermentation process involved. However, it is commercially available on the market at approximately IDR 70,000 per liter. In this study, we required only 20 mL of kombucha per kilogram of feed, making it an economically feasible additive. Combining kombucha with a high-protein diet can be recommended to boost the productivity of catfish farming. However, kombucha can also be added to low-protein feed to optimize fish growth performance. It is also an excellent solution for catfish growers struggling with the price of protein-rich feeds. In conclusion, the addition of kombucha might be a suitable strategy to optimize nutrient uptake and improve fish growth, especially in striped catfish farming.
Conclusion
The present study demonstrated that kombucha supplemen-tation of 20 mL/kg feed, along with higher dietary protein levels, significantly improved growth performance, feed efficiency, and nutrient retention in striped catfish (P. hypophthalmus). The probiotic properties of kombucha, including its beneficial microorganisms like LAB, improved intestinal microbiota and morphology, further enhancing nutrient uptake. Despite the superior growth and nutrient retention observed in fish fed a high-protein diet, kombucha supplementation in low-protein diets also resulted in a significant improvement in fish growth performance, providing a cost-effective strategy for optimizing fish farming. As a result, kombucha supplementation offers a promising solution for improving the growth and feed efficiency of striped catfish. This approach is particularly well-suited to farmers aiming to reduce feed costs while maintaining fish productivity through the use of lower-protein diets.