Background
Olive flounder is a popular seafood and a commercially important fish in Korea; it accounted for about 46.2% of the total cultured fish domestic production of 37,238 tons in 2018 (KOSIS 2018). However, increased infection rate, stunted growth, and decline in the capability to maintain homeostasis and necessary immunity are reasons why the commercial production of this fish has resulted in economic losses. These problems are associated with poor water quality and stress caused by high-density culture to meet the excessive demand for this fish (Maule et al. 1989; Kang et al. 2007).
Most domestic fish farms of olive flounder are set up by using running water at the natural water temperature (Kim et al. 2015). However, large water temperature fluctuations during summer and winter are unsuitable for the optimum growth of olive flounder; consequently, this puts the fish under stress (Barton and Iwama 1991). Stress increases the level of cortisol and glucose in fish (Davis and Parker 1990; Demers and Bayne 1997) and weakens the immune system by reducing the activities of complement, lysozyme, etc. (Sunyer and Tort 1995). The butaphosphan and cyanocobalamin aquaculture drug mixture (BPC) is being used in domestic fish farms to reduce stress and improve growth and immunity and, thus, to reduce the negative effects of the environment. BPC has been used widely in the livestock industry (e.g., for the production of cattle and pigs) and reported to improve liver function, tissue regeneration, and absorption of nutrients by increasing hematogenesis (Flasshoff 1974) and reduce stress by lowering the cortisol concentration (Deniz et al. 2009). Reduced cortisol levels in fish help to maintain normal immune parameters (Seo et al. 2020), and even under high-density culture stress, a reduction in the levels of IL-B, AST, and ALT helps to prevent loss of immunity function and tissue damage (Mechesso et al. 2019). However, research on the effects of BPC in fish is scant.
BPC is regarded as safe and is free of any residual and toxic components (MFDS 2013; EPMAR 2014). However, there have been reports drawing a correlation between BPC administration and tissue damage and mortality in fish. It is possible that the dose used at some fish-farming sites is higher than that recommended. However, to get more clarity, we conducted this study to obtain relevant safety and efficacy data of BPC administration in the cultured olive flounder.
Methods
Olive flounders (Paralichthys olivaceus) with no history of disease or infection were purchased from a local fish farm in Jeju Island. Fish were moved to the Marine Environment Research Institute of Jeju National University and were acclimatized for 1 week to the experimental environment (Fig. 1).
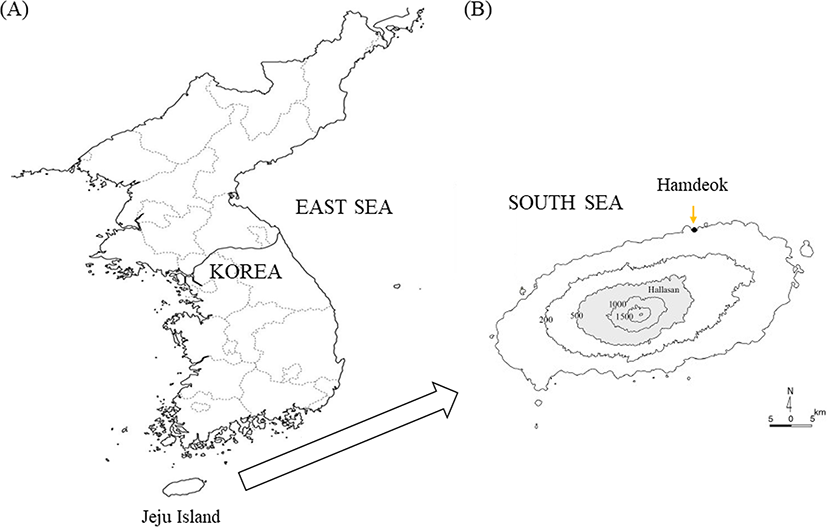
The photoperiod was maintained at 12 h using fluorescent lamps. The temperature of seawater used for culturing was maintained at 20–23 °C. Fish were fed standard food (approximately 3% of the body weight) twice daily at 9 am and 6 pm.
Five fish were selected at random from the control and BPC groups. The fish were anesthetized using 2-phenoxyethanol solution (100 ppm). Blood samples were taken from the tail vein using a heparin-treated syringe and separated by centrifugation (5000 rpm for 10 min). Immunity and stress markers were quantified in the blood and serum. Each analysis was performed in triplicate.
BPC mixture, approved by the National Institute of Fisheries Science’s as an abdominal and intramuscular injection aquaculture drug (butaphosphan 100 mg and cyanocobalamin 0.05 mg/mL), was used at the recommended dose (0.5 mL/kg of fish body weight).
Olive flounders (n = 180; average weight 20.5 ± 1.1 g) were placed into six (30 fish each/three petitions per group) 120-L water tanks containing seawater and grouped as follows: control group intramuscularly administered 0.01 mL of phosphate-buffered saline and test groups intramuscularly administered BPC.
The feeding, behavior, branchial movement, internal and external features, and mortality were evaluated daily until day 14 after BPC administration. Immunity status was evaluated on day 7 and 14 after BPC administration and was based on the levels of lysozyme (LZM), nitro-blue tetrazolium (NBT), superoxide dismutase (SOD), myeloperoxidase (MPO), glutathione peroxidase (GPx), anti-protease (AP), and the total immunoglobulin (TIg) analyses.
Serum LZM activity was determined by a turbidometric assay (Yeh et al. 2008) utilizing lyophilized Micrococcus lysodeikticus cells (Sigma, USA). Briefly, each well of an ELISA plate was filled with 10 μL of serum from individual fish, mixed with 200 μL of an M. lysodeikticus suspension (0.2 mg/mL in 0.05 M sodium phosphate buffer [pH 6.2]), maintained at 25 °C, and the absorbance measured at 530 nm after 1 and 6 min using a plate reader. Each unit of LZM activity corresponded to a 0.001/min decrease in sample absorbance.
The activation of serum macrophages was determined by measuring the neutrophil oxidative-radical generation using the method of Kumari and Sahoo (2005). Samples of whole blood (50 μL) and NBT solution (0.2%) were placed in a glass test tube, to which 1 mL of dimethyl formamide was added to reduce the generated formazan. After centrifugation at 2000Íg for 5 min, the extent of NBT reduction was determined by measuring the absorbance of the supernatant at 540 nm (Genesys 10UA, USA). Dimethyl formamide was used as the blank.
Serum SOD activity was quantified using the SOD assay kit (Sigma, 19160). Samples (20 μL) and 20 μL of WST working solution were placed in a 96-well microplate, 15 μL of the enzyme solution was added, the mixture was diluted with 2.5 mL of the dilution buffer, and the content was mixed. After incubation for 20 min at 37 °C, the absorbance was measured at 450 nm with a microplate reader (Thermo, USA). The results were calculated using the formula
Serum MPO activity was determined using the method of Kumari and Sahoo (2005). Each well of a 96-well plate was filled with 80 μL of Hanks balanced salt solution and 20 μL of serum, 20 mM of 3,3′,5,5′-tetramethybenzidine, and 5 mM H2O2 were added. After a reaction time of 2 min, then 15 μL of 4 M H2SO4 solution was added before the absorbance was measured at 450 nm using a microplate reader (Thermo, USA).
Serum GPx activity was measured following the instructions of the GPx activity kit (Biovision, Inc., Milpitase, CA, USA). Serum samples were placed into a 96-well plate; solutions of NADH, glutathione, and glutathione reductase were added; and absorbance was measured at 340 nm using a microplate reader.
Serum AP activity was determined using the method of Ellis (1990). Serum samples (20 μL) were mixed with 20 μL of trypsin solution and reacted at 22 °C for 10 min. Then, 200 μL of phosphate buffer (0.1 M, pH 7.0) and 250 μL of azocasein (2%) were added to each sample, and the mixtures were reacted at 22 °C for 30 min. The samples were centrifuged at 6000Íg, and 100 μL aliquots of supernatants were placed in the wells of a 96-well plate together with 100 μL of NaOH (1 N). The absorbance was determined at 430 nm.
TIg was determined in the serum using the method of Siwicki and Anderson (1993). Immunoglobulins were precipitated with 12% polyethylene glycol (Sigma, USA) and quantified using the standard Bradford assay kit (Sigma C-690).
Olive flounders (n = 120; average weight 20.5 ± 1.1 g) were placed in six (20 fish each/three petitions per group) 50-L water tanks that could be heated or cooled and grouped as follows: control group intramuscularly injected with 0.01 mL of phosphate-buffered saline and test groups intramuscularly injected with BPC. Water temperature was cooled and heated (by 3–5 °C) alternately every 24 h by using cooling (17–19 °C) thermostats and heating (20–22 °C) rods, respectively. Cortisol and glucose levels were determined on day 1 and day 7. The study was conducted for 7 days only since the possibility of olive flounders dying because of the changes in water temperature needed to be considered; hence, the fish mortality rate was also observed.
Olive flounders (n = 240; average weight 20.5 ± 1.1 g) were placed in 12 (20 fish each/three petitions per group) 100-L water tanks containing seawater and grouped as follows: control group intramuscularly injected with 0.01 mL of phosphate-buffered saline and test groups intramuscularly injected with 0.01 mL, 0.02 mL, and 0.04 mL of BPC. Histological analysis was conducted to evaluate the effect of an overdose of BPC on day 7 and day 14 after administration using five randomly collected fish from each group.
The liver, kidneys, spleen, gill, intestines, and muscles (injected area) were extracted from fish, fixed in Bouin’s solution for 24 h, and dehydrated using 70% EtOH. Tissues were embedded in paraffin (Leica EG 1150HC, Germany) using an embedder (Leica Jung 820, Germany), sliced using a microtome, attached to glass slides, dried, and stained with hematoxylin and eosin. Microscopic examination of these tissues was carried out using an optical microscope (Zeiss LT60, Germany) to observe any abnormal histological changes that may have been caused by BPC.
Results
The safety of BPC was tested in comparison with a control group. No increased mortality or abnormal findings in food intake, internal/external features, and branchial movement were seen (data not shown). LZM, NBT, SOD, MPO, and TIg values, except for GPx and AP activity, were higher in the BPC group than in the control group in the first week, but no significant differences were observed except for the LZM activity (Table 1). The values of all these immunity markers were higher in the second week than in the first week, with higher values being observed in the BPC group than in the control group for all markers. Significant differences were observed in NBT, MPO, and SOD activities between the two groups (Table 1).
Values are mean ± SE (n = 3)
LZM lysozyme, NBT nitro-blue tetrazolium, SOD superoxide dismutase, MPO myeloperoxidase, GPx glutathione peroxidase, AP anti-protease, Tig total immunoglobulin
*Significant difference between the control group and the BPC group based on the t test (P < 0.05)
Significantly lower levels of cortisol and glucose were found in the BPC group than in the control group (Fig. 2). Fish deaths associated with changes in seawater temperature occurred from day 5 in the control group and reached 20% mortality on the last day of the test, whereas no mortality was observed during the same period in the BPC group (Fig. 3).
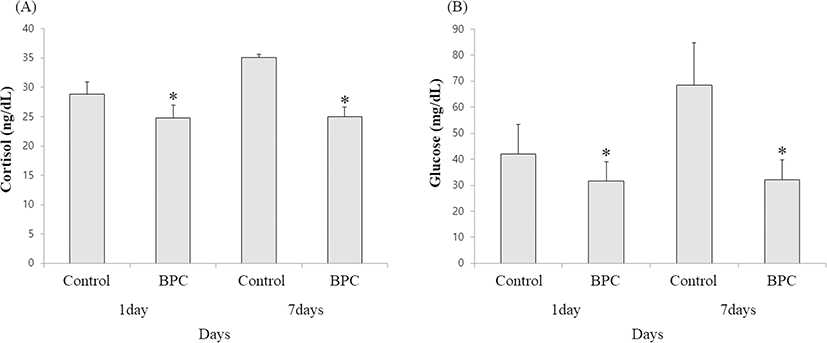
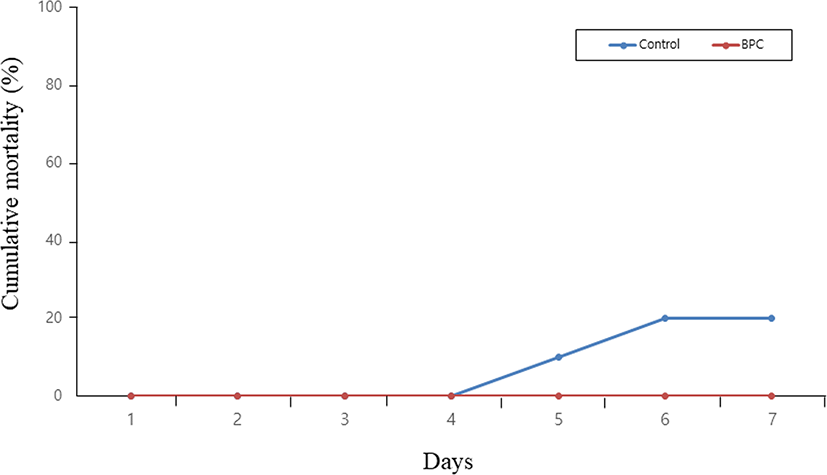
During the 14 days of the study, no abnormality or mortality was observed in any of the groups (data not shown). Histological analysis showed similar results for all the BPC groups. The gill plates and gill filaments showed a constant configuration, and no signs of toxicity, such as bleeding, congestion on gills, or necrosis of epithelial cells, were observed in any of the test groups. No fish showed abnormalities in the muscle injected with BPC, and while minor degeneration was seen in the muscle fiber, similar minor degeneration was observed even in the control group. No bleeding or infiltration of inflammatory cells was observed. No toxic effects such as misalignment of liver cells due to damage to the hepatic cord or degeneration of the tubular epithelial cells in the kidneys caused by the administration of BPC were observed. Red blood cells were observed in the spleen tissue of olive flounders in both the treated and control groups, but no increase in white blood cells was observed. BPC injection did not cause any inflammation or other toxic effects. There were no changes over time to the normal histology after BPC administration (Fig. 4).
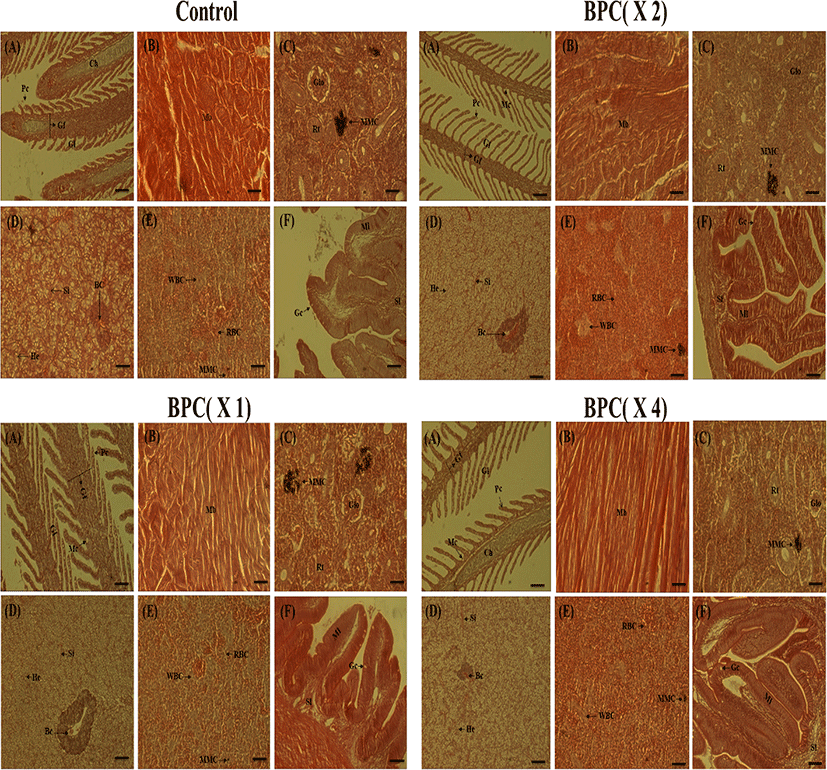
Discussion
BPC is used in domestic fish farms of olive flounder as a registered aquaculture drug to improve immunity and reduce stress in fish (Mechesso et al. 2019; National Institute of Fisheries Sciences 2018; Seo et al. 2020). Although BPC is shown to be effective based on its application in the livestock industry (Pereira et al. 2013a, 2013b; Tabeleao et al. 2017), its effects on fish remain unclear. In this study, BPC was intramuscularly injected into olive flounders at the current recommended dose, and tests were conducted to determine the effects of BPC on immunity and stress. Furthermore, the toxic effects of BPC overdose (1-, 2-, and 4-fold higher than the recommended dose) were studied based on histological examination.
An increase in non-specific immune response reduces mortality and improves fish health (Ji et al. 2007; Yuan et al. 2007; Citarasu 2010). LZM is a widely distributed enzyme that plays a major role in the defense mechanism of higher animals as it functions as an opsonin and protects against microbes (e.g., viruses and bacteria) and disease conditions (e.g., cancer) (Jollès and Jollès 1984). NBT increases reactive oxygen species (ROS) formation by hydrolyzing invading organisms such as viruses and bacteria. MPO plays a role in the inflammatory response; it induces toxicity in the deceased host when destroying the invading organisms (Palić et al. 2005). Activation of SOD and GPx antioxidant enzymes is known to prevent ROS-mediated cell damage (Lopes et al. 2001). TIg improves immunity as it increases acidophilic granulocytes (Picchietti et al. 2007). Our study showed higher immunity in fish treated with BPC than in control fish; this was inferred as all measured parameters improved in the BPC groups whereas only one the control group that improved in the second week. Hasi et al. 2005a, 2005b reported that butaphosphan improved humoral and cell-mediated immune responses in mice; we observed the same in the present study in fish. We observed that fish treated with BPC showed excellent feeding behaviors than control fish when similar feed amounts were supplied. This result may also have some effect on immunity. However, we could not assess the possible action mechanisms of BPC that would promote appetite and immunity; additional tests will be needed to identify such mechanisms.
Factors that could activate stress in fish farms might include physical (selection or transport), chemical (changes in seawater temperature, salinity, or dissolved oxygen), and mental and social stress (high-density culture or sound) (Donaldson 1981). Unlike fish grown in their natural environment, cultured fish may always be exposed to stress as they are cultured in a closed and limited space (Pickering 1990).
Fish cultured under stress for a long time may have increased disease incidence owing to their decreased ability to maintain homeostasis, lesser resistance to stress, and lower immunity, all of which contribute to increased mortality (Heo et al. 2002; Yang et al. 2016).
This study adopted the anesthetic approach suggested by Wer et al. (2009), which involved limiting the time under anesthesia to 3 min and the recovery time to 5 min to suppress a possible long-term physiological stress due to the applied anesthetic.
We observed significantly lower levels of cortisol and glucose in the BPC groups than in the control group. Seo et al. (2020) tested stress in BPC-treated olive flounders kept under high-density culture conditions and reported that cortisol levels decreased sharply in the BPC group compared to those in the control group (phosphate-buffered saline-treated fish); the results of our study are similar. BPC is an amino acid phosphonic acid derivative; while its exact mechanism of action has not been elucidated (EMEA 1999), it was reported that it can accelerate the production of ATP by supplying inorganic phosphate to support oxidative phosphorylation that is an important metabolic process in ATP generation (Hasi et al. 2005a, 2005b). Seo et al. (2020) reported that ATP concentration in olive flounders that received an intramuscular injection of BPC increased compared with that in the control group (phosphate-buffered saline-treated fish). It is likely that the increase in ATP production contributed to the stress reduction by compensating for the stress-induced energy loss; elucidation of the exact underlying mechanisms here would be of interest.
Hematological factors are used as indicators of physiological stress in fish in response to changes in water temperature (Adam 1990; Cataldi et al. 1998). Glucose is one of the factors that, via a secondary reaction, increases cortisol level; increased blood glucose acts as an energy source and compensates for the changes in energy metabolism dynamics that occur when fish are under stress (Barton and Iwama 1991). We determined whether the reduced glucose concentration in the BPC group was related to the energy consumed under stress. Although our results match those by Chang et al. (2001) and show a close correlation with the decreased cortisol levels, further studies might be beneficial to elucidate the mechanism connecting stress, glucose, cortisol, and BPC.
The permitted residual quantities of veterinary drugs used in domestic animals are determined by combining toxicological evaluation and permitted residual quantity evaluation considering international standards. However, butaphosphan and vitamins are classified as safe and are exempt from the evaluation of the residual quantity and toxicity limits (MFDS 2013; EPMAR 2014).
Aquaculture drugs used in fish farms are excreted by or disappear naturally in fish if the dose and usage comply with the existing standards. This can ensure the safety of sea foods. However, some fish farms tend to administer drugs in excessive amounts over the recommended dose. We conducted histological analysis after BPC was administered at 1-, 2-, and 4-fold higher than the recommended dose to determine its safety. The results showed that BPC did not alter the histological findings and that it was safe for olive flounders even at a dose 4-times higher (0.04 mL) than the recommended dose. No side effects were observed with the high dose of BPC.
Seo et al. (2020) reported that the AST level significantly increased within 24 h after injecting 0.3 mL/kg BPC into olive flounders without any toxicity. Histological examination in this study showed normal liver tissues, which is a natural consequence of a long-term investigation as the drug is excreted from the body or disappears naturally over time with no complications seen histologically.
The results of our study indicate that intramuscular injection of BPC improves the immunity of, and reduces stress in, olive flounders. The relationships between immunity and appetite and between immunity and stress are still to be elucidated. In addition, field test data on BPC use will be required.