Introduction
Melanin is one of the major decision factors about hair and body color in vertebrate including human (Fujii 2000). It is synthesized in the melanosomes of melanocytes and affects skin and body color. Melanin cells receive external signals, such as from hormones and ultraviolet rays, that vitalize receptors. Oxidation of tyrosine is increased in melanosomes and the resulting tyrosinase induces melanin synthesis in the melanosomes (Seiji et al. 1963; Korner and Pawelek 1982; Kobayashi et al. 1994). Melanin transports through the dendrites of melanin cells to the skin’s keratinocyte layer. Melanin protects skin tissues from cell deformation and destruction by UV rays (Sulaimon and Kitchell 2003; Costin and Hearing 2007; Kondo and Hearing 2011).
In marine animals, melanin has a protective function against ultraviolet rays, in that it protects cells against damage and stress (Adachi et al. 2005; Suzuki et al. 1994). The olive flounder (Paralichthys olivaceus) and octopus (Octopus vulgaris) have the ability to change skin color and camouflage themselves in response to predators and stress (Fujimoto et al. 1991; Fujii 1993; Hanlon 2007). Melanin in fish has numerous functions including, among others, skin protection, pigmentation, immunity, and stress responses (Amiya et al. 2008; Kittilsen et al. 2009; Sköld et al. 2013; Cal et al. 2017).
Pro-opiomelanocortin (POMC) is usually expressed in the pituitary gland and functions like many peptide precursors, including alpha-melanocyte-stimulating hormone (α-MSH), adrenocorticotropic hormone (ACTH), and β-endorphin (β-END). Melanocortin has five receptors from melanocortin 1 receptor (MC1R) to melanocortin 5 receptor (Cone 2006; Ducrest et al. 2008; Cerdá-Reverter et al. 2011; Ju et al. 2018). MC1R is involved in melanin synthesis and body color. MC2R is the receptor of ACTH and is involved in immune function. MC3R is involved in appetite and homeostasis. MC4R is involved in obesity and priapism, and MC5R is involved in immune regulation, separation of red blood cells, and the maintenance of homeostasis. α-MSH stimulates the synthesis of melanin from melanocytes by revitalizing MC1R. This process darkens skin. The agouti-signaling protein (ASIP) prevents melanin pigmentation on the ventral side of fish by inhibiting α-MSH synthesis which leads to the deactivation of MC1R (Cal et al. 2015). Melanin-concentrating hormone (MCH), which is secreted by the brain, changes body color radically by controlling the cohesion and dispersion of melanin in the chromophore (Baker et al. 1995). It performs various other functions related to appetite and immunity (Takahashi et al. 2004; Matsuda 2009).
According to the Food and Agriculture Organization of the United Nations (FAO), the global production of grouper in aquaculture was 155,000 tons in 2015. The major producing countries are China, Taiwan Province of China (PoC), and Indonesia. These countries contribute 92% of the total production of grouper (Rimmer and Glamuzina 2019). Numerous grouper species studies have been performed on larval development stages as well as the growth and reproductive characteristics (Chauvet 1988; Song et al. 2005; Kim et al. 2013; Lee et al. 2014; Lee et al. 2020).
Serranidae have high industrial value in the domestic aquaculture industry, and some studies (growth (Cho et al. 2015), maturity (Lee et al. 2020), development (Song et al. 2005), digestive physiology (Hur et al. 2013), etc.) are being performed for the development of aquaculture technology. However, research on Serranidae was still rare.
The body color of farmed fish tends to decline in quality compared to wild fish (Booth et al. 2004). This is problematic because it reduces the commercial value of farmed fish. This problem also applies to many farmed grouper species, including the red spotted grouper Epinephelus akaara. Therefore, in order to improve the commercial value of farmed red spotted grouper, research on grouper coloration is required. The coloration of fish provides ecological and physiological information and is a decisive factor in their production value (Paripatananont et al. 1999; Kalinowski et al. 2005; Wang et al. 2006). In our study, we aimed to understand whether rearing red spotted grouper under different light intensity conditions affected their body color. In order to achieve this, we reared fish under different light intensity conditions and searched for the presence of melanin-related gene expression and changes in body color brightness.
Materials and methods
The fish, the artificially produced red spotted grouper was provided by the CR co. ltd (Republic of Korea) and moved to the Marine Science Institute, Jeju National University (Republic of Korea). Before the experiment, fish was acclimatized under natural photoperiod and water temperature conditions and fed to satiation the commercial pellets (Daebong lf fishery association Co., Jeju, South Korea) twice a day (09:00 and 17:00) for a week. All experiments were performed in compliance with the Animal Care and Use Committee guidelines of laboratory animals in the Republic of Korea (Borski and Hodson 2003).
For analysis of tissue specific expression of melanin-related genes, adult red spotted groupers (n = 3, total length 245.3 ± 9.7 cm, body weight 25.0 ± 0.5 g) were taken from the aquarium tank and anesthetized with 0.01% 2-phenoxyethanol (Junsei Chemical Co., Tokyo, Japan) before sampling. After anesthetization, all tissues were extracted and divided into central nervous tissues (telencephalon, tectum, cerebellum, hypothalamus, medulla oblongata, and pituitary gland) and peripheral tissues (retina, tongue, heart, kidney, stomach, intestine, liver, gonad, fin, skin, and muscle). Extracted tissues were immediately frozen using liquid nitrogen and stored at − 80 °C until analysis by real-time qPCR could be completed.
To investigate changes in melanin formation and melanin-related genes in the larval development stage, fertilized eggs were obtained from mature females and males. The fertilized eggs were transferred to individual tanks and allowed to hatch. Fish larvae were reared under natural photoperiod and natural water temperature conditions (24.0 ± 0.5 °C). After hatching, fish larvae were sampled at 0, 1, 2, 3, 5, 7, 10, 14, 20, 25, 30, 35, 40, 45, and 50 days after hatching (DAH). Samples were divided for genetic analysis and microscopic observations. Melanin distribution was observed using an optical microscope (BX43, Olympus Corporation, Tokyo, Japan). For real-time qPCR analysis, collected samples were immediately frozen in liquid nitrogen and stored at − 80 °C.
In order to investigate changes in melanin-related genes’ expression according to light intensity, we performed a fish rearing experiment under different light conditions. Sixty DAH (n = 200, total length 3.2 ± 0.2 cm, body weight 0.34 ± 0.1 g) larvae were reared. Fifty fish were randomly selected and divided from each aquarium tanks (90 L) and kept for 5 days under natural water temperatures and photoperiod conditions before the start of the experiment. Four light intensity conditions (0 lx, 200 lx, 1000 lx, and 2000 lx) were regulated using a photometer, and light source used was LED lights. All experimental groups were reared under a 12 h light to 12 h dark (12 L:12D) photoperiod using a timer under natural water temperature conditions (24.6 ± 0.4 °C) for 9 weeks. The fish was fed commercial pellets (Daehan co., MP3, Busan, South Korea) twice a day during the experiment. Sampling was performed at 3-week intervals, a total of three times, on five fish from each tank. The fish was anesthetized using 0.01% 2-phenoxyethanol, their weight was measured, and the entire length of skin and whole brain were extracted, including the pituitary gland. To analyze the body color difference between all experiments, the fish was photographed using a camera (F90X, Nikon, Tokyo, Japan). Following sampling, samples were immediately frozen in liquid nitrogen and stored at − 80 °C until the real-time qPCR analyses could be performed.
For total RNA extraction, a RiboEx™ (GeneAll Biotechnology, Seoul, Korea) solution was added to the brain, pituitary gland, and skin tissue samples and homogenized using a homogenizer. Total RNA was extracted according to the manufacturer’s protocol and treated using diethyl pyrocarbonate-processed H2O (DEPC H2O) to cleanse and dissolve the total RNA pellet. Total RNA was measured using Nano Vue (GE Healthcare, Buckinghamshire, UK) for cDNA synthesis. In the experiment, total RNA was synthesized using the RQ1 RNase-Free DNase Kit (Promega, Madison, WI, USA). The PrimeScript™ 1st strand cDNA synthesis Kit (Takara Bio Inc., Otsu, Japan) was used for cDNA processing. To produce a species-specific primer for real time-qPCR, partially sequenced melanin-related genes were used (Table 1). Real-time qPCR was performed with 0.2 μL of cDNA using an Evagreen premix PCR kit (ABM, Richmond, BC, Canada). Real time-qPCR was performed with an initial denaturation at 95 °C for 10 min after a reaction at 95 °C for 15 s and 60 °C for 1 min using a CFX96™ real-time system (Bio-Rad Laboratories, Hercules, CA, USA). Expression of genes in each experiment was normalized to amount of the internal control β-actin gene.
The RGB color code for the body color change in fish by light intensity was analyzed by obtaining the average color of an image. A color code was extracted from each image (of equal area) using a free online program Get average color of image by Matthias Klein (Klein 2018).
The experimental results were analyzed with a one-way analysis of variance (ANOVA) using SPSS version 21 (SPSS Inc.) and tested using Duncan’s multiple range test (Duncan 1955) to confirm significance. Values are shown as means ± standard deviations. Statistical significance was determined at a 95% level (P < 0.05).
Results
We aimed to detect expression of MCH, POMC, MC1R, and ASIP mRNAs in the red spotted grouper’s central nervous and peripheral tissues. MCH mRNA expression was significantly higher than the other tissues in the diencephalon (P < 0.05) (Fig. 1a). POMC mRNA expression was significantly higher than the other tissues in the pituitary gland (P < 0.05) (Fig. 1b). MC1R mRNA showed significantly high expression, especially in pelvic fin tissues (P < 0.05) (Fig. 1c). The expression of ASIP mRNA was significantly higher in the tongue and ventral skin (P < 0.05) (Fig. 1d).
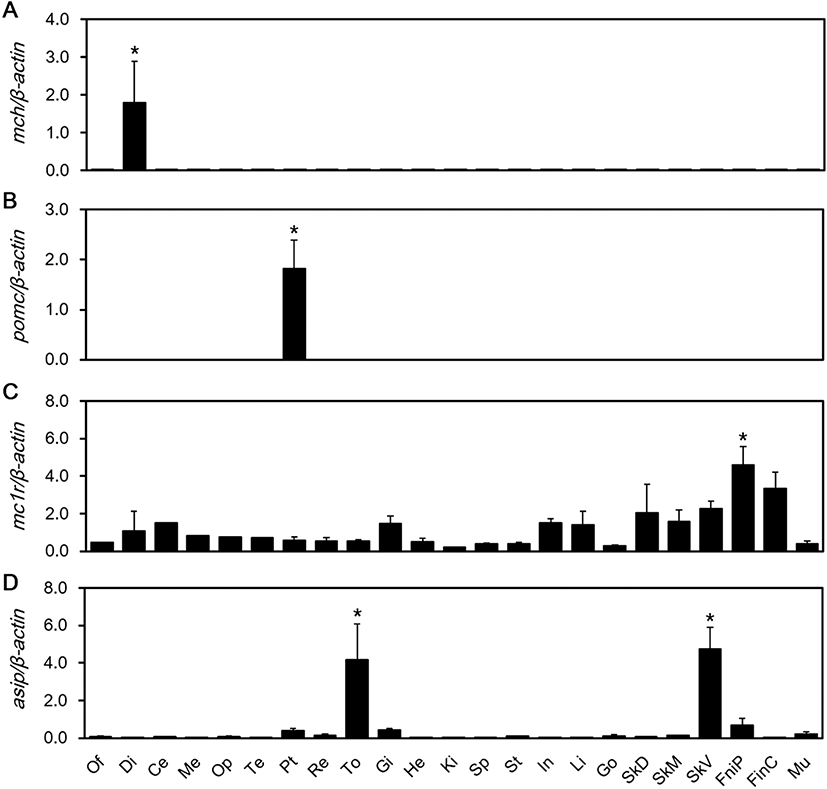
We investigated melanophore formation changes according to development stage in red spotted grouper larvae. At 0 DAH, melanophores were not observed (Fig. 2a). At 1 DAH, a melanin line was observed outside each retina (Fig. 2b). At 2 DAH, melanin had begun to spread from the melanin line outside each retina (Fig. 2c). At 3 DAH, the whole retina of each larva was covered by melanophores and more melanophores had begun to develop at the primordial fin fold and abdominal cavity of each larva (Fig. 2d). At 5 DAH, melanophores in the abdominal cavity were spread out to the vertebra of each larva (Fig. 2e). At 10 DAH, the distribution range of the vertebral melanophores had widened in each larva (Fig. 2f). At 14 DAH, the abdominal cavity of each larva was completely covered by melanophores (Fig. 2g). At 20 DAH, melanophores were observed in the caudal fin of each larva (Fig. 2h). At 25 DAH, melanophores were distributed at the edges of the dorsal fins, and an increase in melanophores was observed at the caudal fins in each larva (Fig. 2i). At 30 DAH, melanophores from the caudal fin began to develop round to the center and sides of each body (Fig. 2j). At 40 DAH melanophores had developed at the dorsal fin and at the edge of dorsal and lateral lines of each larva (Fig. 2k). At 50 DAH, melanophores were observed at the dorsal fin and over the whole body (Fig. 2l).
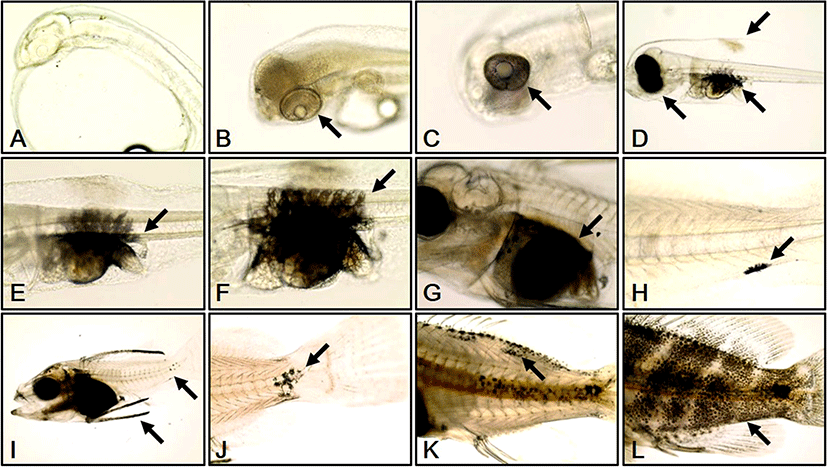
Expression changes of MCH, POMC, MC1R, and ASIP mRNAs according to development stage were observed in red spotted grouper. MCH mRNA expression increased at 3 DAH, was at its significantly high levels at 5 DAH (P < 0.05), then decreased radically thereafter (Fig. 3a). Expression of POMC mRNA increased at 3 DAH, was at its significantly high levels at 5 DAH (P < 0.05), and then decreased until 14 DAH where it remained at low levels of expression (Fig. 3b). Expression of MC1R mRNA was significantly high until 5 DAH (P < 0.05), then it decreased until 25 DAH where it remained at low levels thereafter (Fig. 3c). The expression of ASIP mRNA started by increasing slowly until 10 DAH and significantly high levels at 10 DAH and 20 DAH (P < 0.05). Then, it decreased steadily until 50 DAH (Fig. 3d).
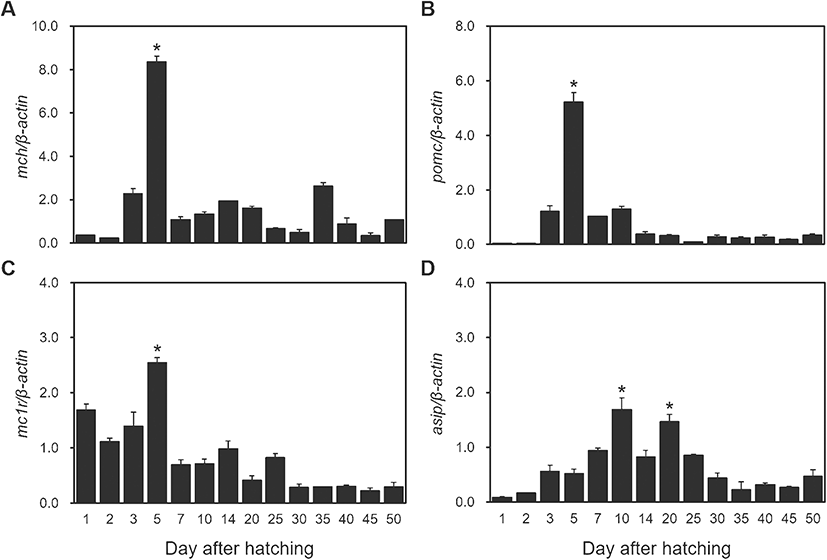
At 6 weeks, the expression of MCH mRNA was at its highest level at 2000 lx and its lowest level at 0 lx (P < 0.05). However, at 9 weeks, there was no significant difference between the experimental groups (Fig. 4a). At 6 weeks, the expression of POMC mRNA was highest at 200 lx, and at 9 weeks, the highest level was confirmed at 0 lx. The lowest level of POMC mRNA was confirmed at 1000 lx (P < 0.05) (Fig. 4b). Expression of MC1R mRNA was at its highest level at 200 lx, and at 3 weeks, it was at its lowest at 2000 lx (P < 0.05). However, at 6 weeks, there was no significant difference in the expression of MC1R mRNA between the experimental groups. At 9 weeks, the highest level was observed at 0 lx, and the lowest level was observed at 1000 lx (Fig. 4c). The expression of ASIP mRNA was at its highest level immediately after the beginning of the experiment. It then maintained a low level of expression and showed no significant difference between the experimental groups (Fig 4d). When we measured the RGB color value of the red spotted grouper’s skin, the 0 lx group returned values of 86, 71, and 58 as their darkest colors. The RGB values from the 200 lx group were 88, 71, and 59 and from the 1000 lx group were 137, 116, and 99, in which 1000 lx was the brightest colors. The RGB values of the 2000 lx group were 105, 92, and 81 (Table 2).
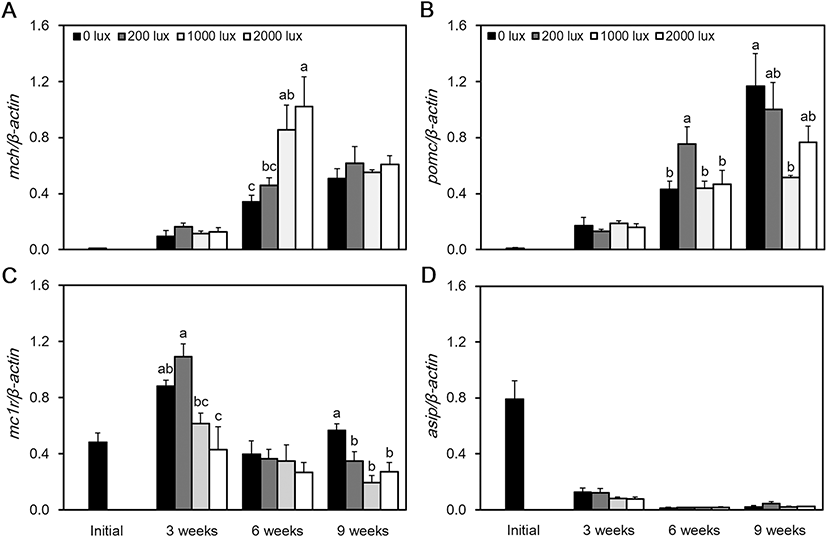
0 lux | 200 lux | 1000 lux | 2000 lux | |
---|---|---|---|---|
RGB (Red, Greed, Blue) | 86, 71, 58 | 88, 71, 59 | 137, 116, 99 | 105, 92, 81 |
Discussion
Recently, photobiology studies on fish have been conducted on various adaptive physiological characteristics, including body color, growth, and metabolism (Appelbaum and Kamler 2000; Petrell and Ang 2001). This study investigated the adaptive physiology of red spotted grouper’s melanin-related genes with respect to light intensity. According to our results, the expression of genes related to melanin development in red spotted grouper larvae showed that the expression of MCH, POMC, and MC1R mRNAs began to increase at 3 DAH, with the highest levels observed at 5 DAH. During this period, melanophores completely covered the body and started to form dorsal fin fold and abdominal cavities. These results are considered to reflect the activation of melanin-related genes’ expression from 3 DAH to 5 DAH, which leads to melanin synthesis in the red spotted grouper. ASIP mRNA was highly expressed 7 to 25 DAH, and during this period, the abdominal cavity was covered with melanophores and the outer part of the abdominal cavity was surrounded by iridophores.
The ASIP gene prevents the division and increase of melanin cells and makes skin color brighter (Suzuki 2013). From experimental results on goldfish (Carassius auratus) (Cerdá-Reverter et al. 2005), ASIP mRNA was found to have a genetic homogeny of mammal ASIP mRNA, along with mice, and it plays an important role in forming the ventral color pattern. In goldfish, ASIP mRNA is mainly expressed in ventral skin and has not been detected in dorsal skin. These results suggest that ASIP mRNA is mainly involved in differentiation to iridophores in ventral skin. Therefore, the increased ASIP mRNA expression in red spotted grouper larvae at 7 DAH, that we observed, may stimulate the development of iridophores outside the abdominal cavity.
Melanophores in red spotted grouper larvae specimens were observed at 1 DAH formed outside the iris and shaped in a ring. At 3 DAH, the melanophore was observed, in each specimen, in the dorsal fin fold and abdominal cavity. At 5 DAH, the melanophore was observed at the lateral line in larvae. At 20 DAH, larvae coloration started to develop when the expression of the melanophore was at the end of lateral line. At 40 DAH, the melanophore started to color the whole body and tail fin. The body color development of red spotted grouper larvae showed a similar development pattern as the brown-marble grouper (Epinephelus fuscoguttatus), which is in the same family (Kohno et al. 1993). The color development of brown-marble grouper larvae also starts with observation of melanophores in the eyes, after which it develops chromophores throughout the abdominal cavity, dorsal fin spine, and caudal parts. The melanophore starts to condense at the dorsal fin spine and caudal parts, and the body color develops through the head, tail, dorsal fin spine, and whole body. However, some studies have reported melanophore development stages that differ from our results. In rock porgy (Oplegnathus punctatus), the melanophore can be observed before hatching (Park et al. 2015). The rock porgy’s melanophore pigments move back to the head and surround the myotome throughout the tail at fertilization after 15 h, which is termed organogenesis. When hatched, the melanophore is already developed around the oil drop and covers the body’s entire skin surface. In starry flounder (Platichthys stellatus), before larval melanophore development in the eyes, the melanophore has already begun to develop in the primordial fin and tail (Yamashita et al. 2014). After melanophore development has been completed in the eyes, the melanophore begins to develop throughout the body from the primordial fin fold and body center. Based on these contrasting results, melanophore development patterns are likely to be species-specific.
We investigated melanin-related gene expression in red spotted grouper according to different light intensities. At 6 weeks, MCH mRNA tended to increase its expression levels with an increase in light intensity. However, the expressions of POMC mRNA and MC1R mRNA were highest at 0 lx and lowest at 1000 lx. At the end of the experiment, the expression of MC1R mRNA was at its highest at 0 lx and its lowest at 1000 lx. In case of MC1R mRNA expression, it was significantly higher in dark conditions in the 3 weeks and 9 weeks, and there was no significant difference in the 6 weeks, but in the dark conditions, MC1R mRNA expression tended to be high. However, the expression of ASIP mRNA was at its highest during the initial period of the experiment but maintained a low level of expression afterwards. In terms of body color (RGB cord value), the darkest color value was observed in the 0 lx group, and bright color value was observed in the 200 lx, 2000 lx, and the 1000 lx groups.
A lot of research studies have reported that MCH is closely involved with background color (Yamanome et al. 2005; Chung et al. 2018; Diniz and Bittencourt 2019; Kasagi et al. 2020). To observe morphological color changes in goldfish, fish was reared under different background color conditions and the expression of M1CR, MC5R, MSH, MCH, and ASIP mRNAs was checked (Mizusawa et al. 2018). In the white-reared fish, MCH mRNA expression was higher than that in black-reared fish, and POMC mRNA expression was lower than that in the black-reared fish. In the xanthophores in the scale, MC1R did not always follow the background color change, but MC5R followed the background color change. Mizusawa was suggested that MC5R might be a major factor reinforcing the function of MSH in morphological color change. ASIP mRNA has high expression in the ventral skin but no expression in the dorsal skin. Therefore, MC1R, MC5R, MCH, and MSH were associated with background color adaptation and ASIP mRNA might be not.
Many studies have aimed to determine the effects of light intensity on survival rate, growth, swimming, feeding, coloration, and various other physiological phenomena (Batty et al. 1990; Boeuf and Le Bail 1999; Reichard et al. 2002; Richmond et al. 2004; Luchiari et al. 2006; Liu et al. 2012; Lee et al. 2017). Light can induce melanogenesis and increase the synthesis of the melanophore, which can make the body coloration darker (Odiorne 1957). When the Australian snapper (Pagrus auratus) was reared under different degree of shading conditions (0%, 50%, and 95% shading form incident radiation), the group under the 0% degree of shading conditions showed darker body coloration than the other groups (Booth et al. 2004). In addition, the body color of Chinese longsnout catfish (Leiocassis longirostris) tends to be dark under a strong light intensity, and their survival rate tends to drop under extremely strong light intensity conditions (Han et al. 2005). This study indicates that the light intensity affects melanin synthesis. In these experiments, we conclude that the light sources such as sun or ultraviolet rays stimulate the skin and lead to melanin synthesis for skin protection. And by that result, the body colors became more dark.
Research on body color change in red porgy (Pagrus pagrus) as a result of changes in light intensity has shown that red porgy tends to have bright body coloration beside a white background with a high intensity of light (Pavlidis et al. 2008). After observing body color change in ocellaris clownfish (Amphiprion ocellaris) under 20–50, 600–850, and 2700–3500 lx conditions, specimens were found to have a bright color on their back and tail fins under low intensity light (Yasir and Qin 2009). These results indicate that the body color has adapted to the environment condition, affected by the amount of light. These researches suggest that light intensity can affect body color. Comparing our research results, the bright light intensity is thought to brighten up the body color because of the melanin concentrated due to increased expression of MCH mRNA, and melanin synthesis decreased due to reduced expression of POMC and MC1R mRNA.
In our study on the melanin-related genes of the red spotted grouper, POMC mRNA, MC1R mRNA, and MCH mRNA showed different gene expression patterns under different light intensity conditions. The expression of ASIP mRNA was not affected by light intensity. It is thought that under controlled light intensity conditions, the expression of POMC mRNA and MC1R mRNA induces melanin synthesis. The concentration of MCH mRNA was affected by light conditions. The low expression of ASIP mRNA is most likely due to the development of iridophores in the abdominal cavity as the larvae grew.
Conclusion
The aim of this study is the change in the body color and melanin-related gene expression of red spotted grouper by light intensity. Our results suggested that when light intensity goes stronger, MCH mRNA expressed higher, and POMC and MC1R mRNAs expressed lower. However, ASIP mRNA expression did not changed. With these results, we suggest that MCH, POMC, and MC1R mRNAs are affected by light intensity. Further research is needed to understand the relationship between melanin composition and gene expression in relation to the external environment and body color change. Our study contributes valuable baseline data that advances our knowledge of body color stimulation under environmental conditions in the red spotted grouper.