Introduction
Prostate cancer is the most common cancer among men worldwide and a leading cause of cancer-related deaths in men in Western countries (Quinn & Babb, 2002). Since the incidence rates of prostate cancer have recently increased dramatically in less-developed countries such as in Asia and Africa, which have traditionally had the lowest incidence rates (Baade et al., 2009), the effective treatment of prostate cancer has gained increased attention. Since prostate cancer usually develops slower than other cancers and has a long latency period of more than 10 years before developing into a symptomatic and clinical disease, the incidence of prostate cancer may be reduced by blocking the multi-step process involving the progression from small and low-grade lesions to large and high-grade carcinomas (Lee et al., 2008). However, advanced prostate cancer is more difficult to treat than early prostate cancer because the cancer cells do not respond to most cytotoxic chemotherapeutic agents. Therefore, there is a need for a more effective therapy for advanced prostate cancer. Prostate cancer has been known to be associated with the abnormal growth of cells in the prostate gland (Kyprianou et al., 1996). Many studies have focused on various signaling pathways that regulate the growth and proliferation of prostate cancer cells and on the development of the related chemoprevention agents (Gupta et al., 2003).
Recently, interest in bioactive ingredients from marine fungi has grown because some marine fungal-derived compounds have various biological activities, including control of cell growth (Swathi et al., 2013). Specifically, marine fungi Aspergillus fumigatus has been reported to generate many structurally and biologically diverse secondary metabolites (Finefield et al., 2012), and their derivatives have been mainly studied for their potential use in the treatment of microsporidiosis (Molina et al., 2002) and amebiasis (Killough et al., 1952) and their antiangiogenic properties (Ben-Ami et al., 2009). However, there were few reports on the anticancer activity of compounds from A. fumigatus against cancer. Recently, we reported the isolation of demethoxyfumitremorgin C (DMFTC) from the ethyl acetate (EtOAc) extract of A. fumigatus strain MFS150 and the compound inhibits growth of human prostate cancer PC3 cells (Kim et al., 2017); however, the detailed data regarding to cell cycle arrest related to apoptosis has never been examined. In the present study, we revaluated the antiproliferative activity of DMFTC in human prostate cancer PC3 cells and investigated its functional mechanism on cell cycle progression. The results indicated that this compound induces G1 phase arrest during cell cycle progression and apoptotic cell death.
Materials and Methods
The PC3 human prostate cancer cell line was obtained from the Korean Cell Line Bank (KCLB, Seoul, Korea). RPMI 1640 medium was obtained from Lonza (Walkersville, MD, USA), and fetal bovine serum (FBS) and penicillin/streptomycin were bought from Gibco BRL, Life Technology (Carlsbad, CA, USA ). All antibodies used for the western blot analysis were from Santa Cruz Biotechnology (Santa Cruz, CA, USA). 3-(4,5-Dimethyl-2-yl)-2,5-diphenyltetrazolium bromide (MTT) reagent was obtained from Sigma Chemical (St. Louis, MO, USA). Other chemicals and reagents were of analytical grade and commercially available.
DMFTC was isolated by the previous method described by Li et al. (2014) with slight modifications. We isolated A. fumigatus MFS-150 from surface of marine green algae, collected at 2009 at Seosaeng-myeon, Ulsan, in the Republic of Korea. The fungal strain was cultured at 25°C and stored in 20% glycerol with YPG medium (1% yeast extract, 1% peptone, 10% glucose, 4% agar, 50% seawater, and 50% distilled water). The fungus was cultured (30 L) for 30 days at 25°C and pH 7.6 in YPG medium. The culture broth was extracted (2.3 g) with EtOAc (1:1.5 v/v, 1:1 v/v, broth-EtOAc) twice and was fractionated by silica gel flash chromatography [n-hexane-EtOAc 100%–0% and CH2Cl2-MeOH 1:1] to yield several fractions. The major fraction (1,251.4 mg) was further purified using octadecyl functionalized silica gel (ODS; H2O-MeOH, 100%–100%) with 1 mL/min flow rate and Sephadex LH-20 chromatography (H2O-MeOH, 100%–100%). Finally, we performed high-performance liquid chromatography (HPLC) (YMC ODS-A column; 250 × × 10 mm l.D, S-5 μm, 12 nm, methyl alcohol [MeOH]) to isolate DMFTC (final 7.2 mg). The structure and molecular formula of DMFTC, compared to previous data (Kim et al., 2017), were ascertained from 1H, 13C NMR (nuclear magnetic resonance), and low resolution electron impact mass spectrometry (LREI-MS) data. LREIMS m/z: 349 [M]+ (C21H23N3O2).
PC3 human prostate cancer cells were grown in 10% FBS-containing RPMI 1640 media and incubated at 37°C in 5% CO2. In the early less than passages 5, the cell growth inhibition and cell death effects of the compounds from A. fumigatus on PC3 cells were analyzed using MTT [3-(4, 5-dimethyl-2-yl)-2, 5-diphenyltetrazolium bromide] assays. To examine the anti-proliferative effect of DMFTC, the cells seeded were incubated for 24 h in 96-well plates at a concentration of 5 × 104 cells/mL. The cells were treated with different concentrations of the compounds (0, 25, 50, and 100 μM). At the end of the incubation time, 100 μL MTT solution (1 mg/mL in DMSO) was added to well and incubated for another 3 h. The formed formazan salt was dissolved in 100 μL DMSO, and the absorbance was measured at 550 nm (GENiosr microplate reader, Tecan, Grödig, Austria). The data were described as means ± SD of at least three independent assays.
We performed cell cycle analysis of propidium iodide (PI)-stained nuclei using CellQuest software on a FACS Calibur flow cytometer (Becton-Dickinson Biosciences, Heidelberg, Germany). PC3 cells were cultured in 6-well plates at a density of 5 × 104 cells/mL and then were treated with DMFTC after 24 h for 72 h at various concentrations (0, 25, 50, and 100 μM). The cells were harvested at each incubation time, pelleted by centrifugation for 5 min at 500 × g, and resuspended in the chilled PBS. The cells were fixed with 70% ice-cold EtOH and stored at 4°C. Prior to analysis, cells were washed again with PBS, resuspended in 1 mL of a cold PI solution with 50 μg/mL RNase A, and further incubated on ice for 20 min in the dark. We analyzed the data using single histogram statistics (Yin et al., 2014).
Cell death was assessed by fluorescent microscopy after staining cells with Hoechst 33258 and PI as expressed by Naito (1998). Cells were grown in 24-well plates at a density of 5 × 104 cells/mL and treated with different concentrations of DMFTC after 24 h, and further incubated for 72 h. The cells were washed with PBS twice and fixed with a 4% formaldehyde solution in PBS for 1 h at room temperature (RT). The fixed cells were washed with PBS three times and were stained with 1 μg/mL fluorescent DNA-binding dye Hoechst 33258 and incubated for 1 h at RT to reveal nuclear condensation/aggregation. Cells were treated with different concentrations of DMFTC for 72 h for PI staining. The DNA-specific fluorescent dye PI 5 μg/mL was added to the culture medium. The plates were additionally incubated for 30 min at 37°C. The Hoechst 33258- and PI-stained cells were visualized and photographed under a fluorescence microscope (Motic AE31, MHG-100B, Jed Pella, Redding, CA, USA; DM3000, Leica, Wetzlar, Germany).
Western blotting was performed according to standard procedures. Cells were cultured at a density of 5 × 104 cells/mL in 6-well plates. The cells were treated with different concentrations of DMFTC for 72 h after incubation for 24 h. Cells were lysed in RIPA buffer at 4°C for 30 min. Total proteins were obtained, and 100 μg/mL of protein was separated using 10% SDS-PAGE and transferred onto a nitrocellulose membrane (Amersham Pharmacia Biotech, Little Chalfont, UK). The membrane was blocked using TBS-T buffer containing 5% skim milk and 0.1% Tween-20 for 1.5 h at RT. The blots were incubated for 1 h with suitable antibodies at 25°C. The respective proteins were confirmed with a chemiluminescent ECL assay kit (Amersham Pharmacia) according to the manufacturer’s instructions. The western blot bands were visualized using a LAS-3000 system and detected using MultiGauge V 3.0 software (Fujifilm Life Science, Tokyo, Japan).
Results
To identify compounds with cytotoxic effect against human advanced prostate cancer cells from marine fungus A. fumigatus, DMFTC was purified from a pool of secondary metabolites. The chemical structure and molecular formula of DMFTC (C21H23N3O2) were ascertained from 1H, 13C NMR, and LREI-MS spectroscopic data together with comparison to the data published previously (Cui et al., 1996, 1997; Kim et al., 2017). The compound was identified as DMFTC, as illustrated in Fig. 1 and Table 1.
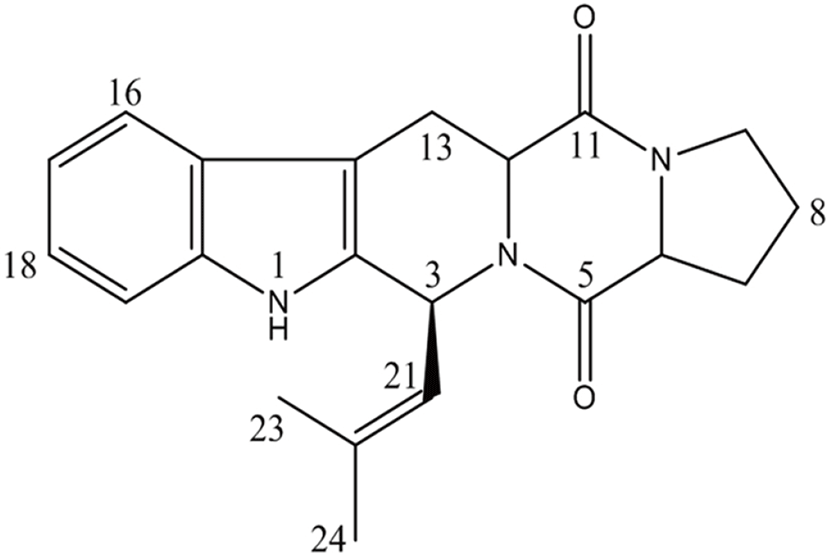
The anti-proliferation activity of the isolated DMFTC was confirmed in PC3 human prostate cancer cells. PC3 cells were treated with various concentrations (0, 25, 50, and 100 μM) of DMFTC for 24, 48, and 72 h. Cell viability at each condition was measured by MTT assay. Fig. 2 shows that DMFTC delayed the growth of viable cells and suppressed the growth of PC3 cells in a dose- and time-dependent manner. The IC50 value of DMFTC was estimated to be 73.35 μM at 72 h.
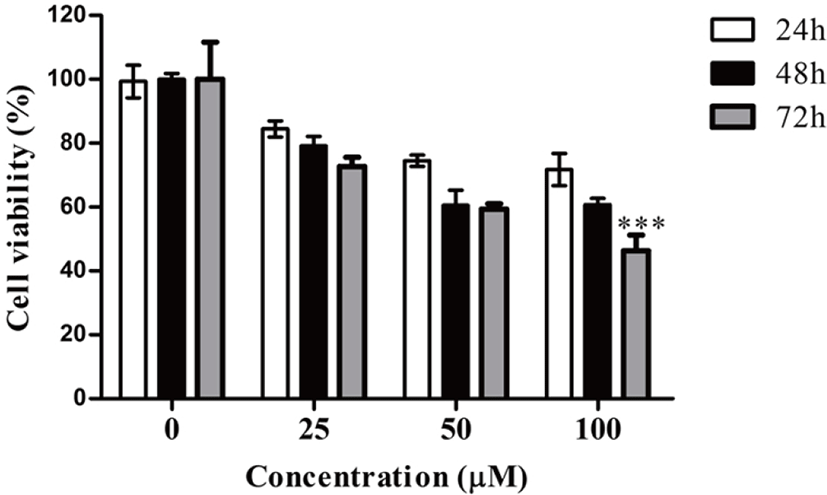
To explore the mechanism responsible for the inhibitory effect of DMFTC on cell growth, we first examined whether DMFTC regulates the cell cycle progression of PC3 cells. PC3 cells were treated with various concentrations (0, 25, 50, and 100 µM) of DMFTC and stained with PI, and the cell cycle progression then was analyzed by flow cytometry. To identify the location of the major cell cycle stage where DMFTC inhibits the cell cycle progression in PC3 cells, the dead cells and debris were excluded. As shown in Fig. 3, the cell cycle progression was arrested in G1 phase when cells were treated with DMFTC. Compared with vehicle-treated control cells, DMFTC treatment increased the number of G1 phase cells and decreased the number of S phase cells in a concentration-dependent manner. In the presence of 100 μM, 70.44% of cells remained in G0/G1 phase compared with 59.77% of cells in the vehicle-treated control group. This result suggests that DMFTC decreases cell cycle progression through cell cycle arrest at G1 phase.
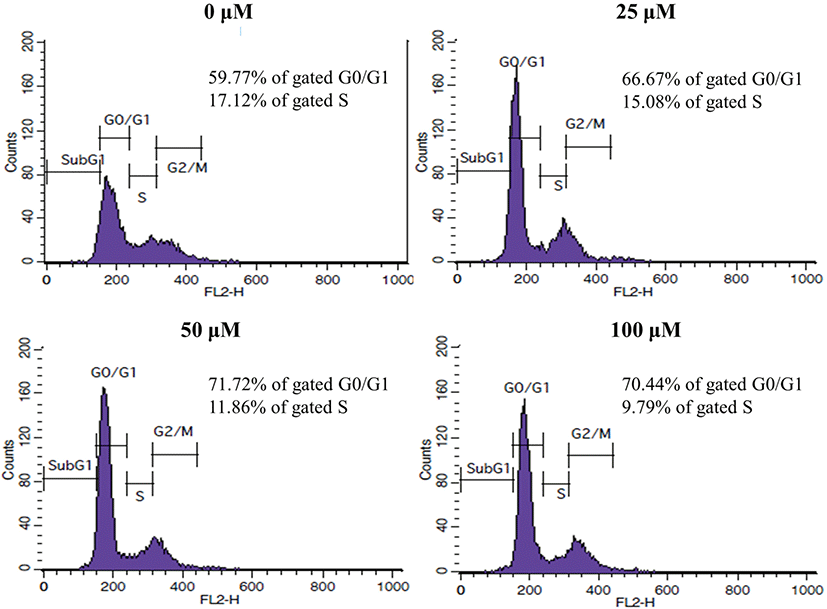
We next examined whether the inhibition of PC3 cell growth by DMFTC is related to apoptotic cell death. Hoechst 33258 and PI were used together for the fluorescence imaging analysis of the apoptotic cells. PI crosses the plasma membrane of cells in the later stages of apoptosis or that are already dead. Fig. 4 shows that DMFTC induces nuclear margination and obvious morphological changes. Late apoptotic cells with fragmented chromatin were also observed. The number and intensity of PI-positive non-viable cells also increased with exposure to DMFTC in a dose-dependent manner. This result indicates that DMFTC induces apoptotic cell death in PC3 cells.
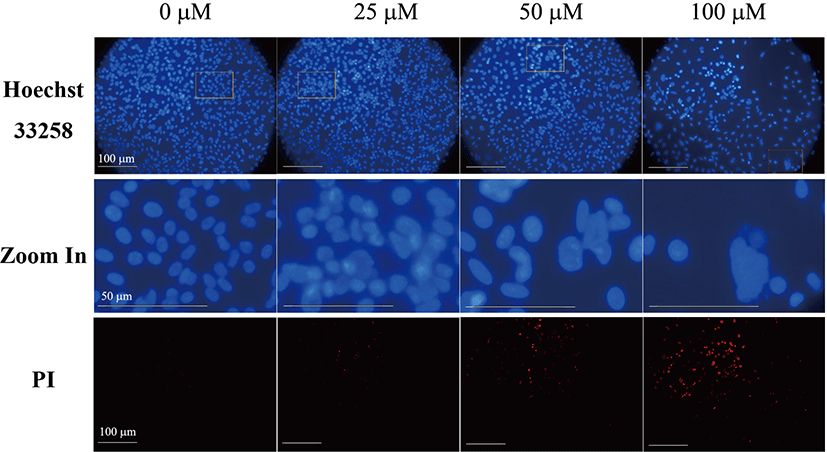
We also analyzed the effect of DMFTC on the expression of cell-cycle regulatory proteins that could be involved in G1 arrest and apoptosis. The tumor suppressor p53 functions an important role in the cell cycle checkpoint system responsible for progression from G1 into S phase (Livingstone et al., 1996). Many chemotherapeutic drugs used to treat cancer increase the level of p53 and activate the protein, leading to G1 phase cell cycle arrest and apoptosis (Bykov et al., 2002; Clarke et al., 1993; Di et al., 1994). The p53-dependent G1 arrest is associated with the activation of p21 and the subsequent inhibition of G1 cyclin-Cdk complexes, such as cyclin D-Cdk4/CdK6 and cyclin E-Cdk2, leading to G1 cell cycle arrest and apoptosis induction (Sherr, 1996). Therefore, we examined whether the p53 and p21 tumor suppressor factors and the downstream cell cycle-related proteins are involved in the anti-proliferative effect of DMFTC. The treatment of PC3 cells with DMFTC induced the activation of p53 and p21. DMFTC treatment increased relative ratio of the intensity of phospho-p53 or phosphor-p21 to total p53 or p21 protein on dose-dependent manner.
The levels of cyclin D, cyclin E, Cdk2, and Cdk4 decreased following DMFTC treatment (Fig. 5). These results indicate that A. fumigatus-derived DMFTC reduces the proliferation of PC3 human prostate cancer cells through p53/p21-dependent G1 cell cycle arrest and the induction of apoptosis.
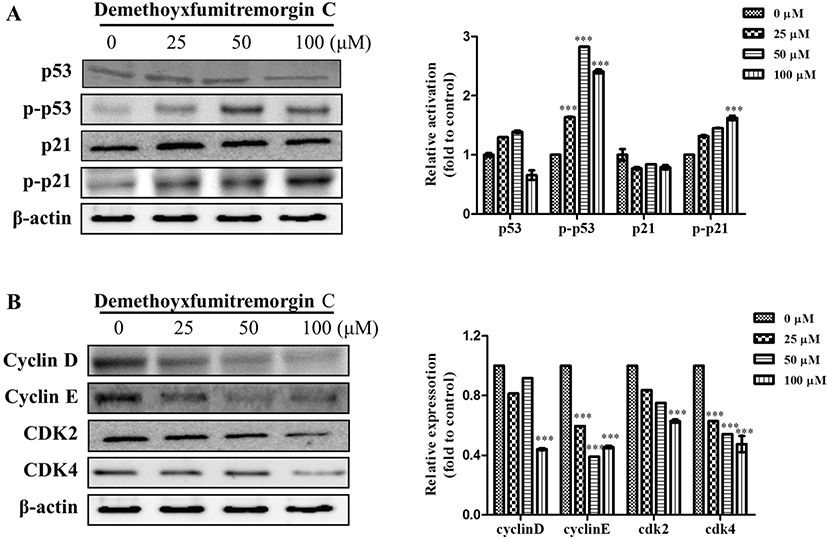
Discussion
The most effective systemic therapy for prostate cancer is androgen deprivation or the removal or blocking of the male hormone testosterone because androgen-dependent testosterone is essential for the growth and survival of prostate cells, whereas androgen deprivation causes the apoptosis of prostate cells (Hussain et al., 2013). However, some men do still develop prostate cancer. Aggressive genetic alterations cause cells to multiply, and these cells then eventually disrupt the internal structure of the prostate by breaking out of the gland and invading the stromal tissue and extending outside the prostate, acquiring advanced metastatic properties. However, in such cases of advanced prostate cancer, unfortunately, the cancer cells become resistant to apoptosis and do not respond to cytotoxic chemotherapeutic agents (Pilat et al., 1998). Therefore, agents that inhibit cell growth and induce apoptotic death in the hormone-refractory prostate cancer cells would be useful for the prevention and treatment of advanced prostate cancer. In the present study, we showed that DMFTC derived from the marine fungi A. fumigatus delays cell cycle progression and induces apoptotic cell death in PC3 advanced human prostate cancer cells. This result supports well our previous data, in which we indicated that DMFTC causes apoptosis as determined by Annexin V/PI staining and decreases mitochondrial membrane potential (Kim et al., 2017). We have also reported that DMFTC inhibits preferentially the proliferation of PC3 cells rather than other human normal and cancer cells lines, including MRC5, Hela, MCF-7, and HepG2 (Kim et al., 2017). Therefore, all results suggest that DMFTC has the potential to be a significant therapeutic mechanism for the prevention and treatment of advanced prostate cancer. DMFTC regulates cell growth by p53/p21-dependent G1 phase cell cycle arrest and apoptotic pathways. In fact, the inhibition of cell cycle progression is an appropriate target for cancer treatment. Many drugs that target the cell cycle have been tested in clinical trials (Cuzick et al., 2011). When a cell needs to stop the cell cycle in G1, kinases such as Chk2 and Chk1 phosphorylate the tumor suppressor p53, which results in the transcriptional activation of p21, an inhibitor of the G1-to-S promoting complex cyclin E-Cdk2 and/or cyclin D-Cdk4 complex, thus keeping the cell from transitioning to S phase (Sherr, 1996). p53 also plays a vital role in cancer cell apoptosis through the regulation of proteins such as the Bax (a cell death inducer) and Bcl-2 (a cell death inhibitor) families (Cory et al., 2003; Karnak & Xu, 2010). Our data show that the inhibition of cell growth and induction of apoptosis by DMFTC in PC3 cells is most likely associated with the role of p53/p21-dependent cyclin-Cdk complexes. Therefore, these results suggest that DMFTC obtained from the fermentation extract of the marine fungus A. fumigatus MFS-150 may supply new therapeutic insights to prevent and treat advanced prostate cancer that is resistant to conventional treatments.
Cui et al. (1996) previously reported that DMFTC isolated from the A. fumigatus strain BM939 exhibits an inhibitory activity on the M phase cell cycle progression of mouse mammary carcinoma tsFT210 cells. The tsFT210 cells are a temperature-sensitive p34cdc2 mutant cell line. In their study, they first synchronized the cells in G2 phase and then released them to screen for G2/M phase inhibitors that trigger M phase-promoting factors related to cdc2 kinase and cyclin B for mitosis. Therefore, the effect of DMFTC on the M phase arrest of tsFT210 cells may be stronger than its effect on PC3 cells in our study. In the assays of randomly cultured PC3 cells in present study, we did not assess M phase cell cycle arrest by DMFTC. This difference in the effect of DMFTC on cell cycle arrest might be due to the different molecular determinants between PC3 human advanced prostate cancer cells and tsFT210 mouse mammary carcinoma cells. More studies may be needed to confirm the anti-proliferation effect of DMFTC in various cancer cells.
Conclusion
In the present study, we found that DMFTC from A. fumigatus MFS-150 inhibits PC3 cells growth through G1 phase cell cycle arrest and apoptosis induction. DMFTC activated the tumor suppressor p53 and the Cdk inhibitor p21, which regulate the cell progression into the G1 phase, whereas decreased the levels of the G1-positive downstream regulators cyclin D, cyclin E, Cdk2, and Cdk4. DMFTC could therefore be considered as a useful agent for treatment of human prostate cancer.