Introduction
Halophytes are salt-tolerant plants which usually grow in coastal salt-marshes containing mixed organic material and mineral salts from land and ocean. The halophytes that inhabit by developing a mechanism in order to adapt to extreme environments (high salinity and strong wind, etc.) are likely to have strong bioactive metabolites, due to which halophytes have attracted increasing attention from researchers in recent years. The Sonchus genus is known more than 50 species. Most of which are annual herbs and many are widespread, invasive and noxious weeds (Kim et al., 2007). In previous studies, Sonchus species have been reported to exhibit anti-tumor, teratogenic, antihypertensive, antioxidant, vasodilatation, anti-inflammatory, antimicrobial, antidiabetic, antinociceptive and xanthine oxidase inhibitory activities. In addition, Sonchus species have been demonstrated to exert inhibitory effects on gastro intestinal motility and locomotor activity (Hendriani et al., 2014; Huyan et al., 2016; Khader et al., 2013; Mawalagedera et al., 2016; Mushtaq et al., 2016; Poudel et al., 2015; Sukandar & Safitri, 2016; Sukandar et al., 2016; Vilela et al., 2009; Xu et al., 2011). Many phytochemicals, such as diterpenes (asperal, emodin), triterpenes (erucaoic acid, oleanolic acid, ursolic acid, lupeol, betulinic acid, α-amyrin, β-amyrin) and flavonoids (luteolin, apigenin, kaempferol, quercetin, luteolin-7-O-β-D-glucoside, isoquercitrin, astragalin) have been reported to be present in Sonchus species (Bai et al., 2008; Elkhayat, 2009; Khan et al., 2014; Muhammad et al., 2012; Xia et al., 2012; Yin et al., 2008). Among Sonchus species, S. brachyotus is a perennial halophyte belonging to the Cichorieae tribe within the Asteraceae family. S. brachyotus grows to 30–100 cm in height and the stem is usually unbranched below synflorescence and glabrous. Its capitulum consists of many florets (170–300), and the peduncle is slender with apical white tomentose. S. brachyotus is mainly distributed in grassy slopes of mountains and alkaline areas, ranging from 300 to 4,000 m altitude, in Korea, Japan, China, Russia, Mongolia, and Kazakhstan. The young leaves of S. brachyotus, which are known to possess diuretic activity, are also used to treat hepatitis and hemorrhage in Korea (Nugroho et al., 2012). S. brachyotus has been reported for exhibiting biological activities, including antioxidant, anti-inflammatory, antibacterial and antidiabetic activities (Lee et al., 2018; Li et al., 2017; Xia et al., 2011). But, many studies have described the isolation and identification of secondary metabolites from Sonchus species, however, investigations of S. brachyotus have been scarce. The aim of this study was to evaluate the antioxidant and anti-inflammatory activities of secondary metabolites isolated from S. brachyotus.
Materials and Methods
S. brachyotus specimens were collected in August 2016 in Ganghwa-gun, Incheon, Korea. These specimens were then identified by Korea Institute of Coastal Ecology (KICE) and deposited at National Marine Biodiversity Institute of Korea (MABIK NP60150005).
The dried whole plant of S. brachyotus (1.54 kg) was lyophilized and then pulverized. The pulverized S. brachyotus was extracted with 70% EtOH (4 L × 8) and evaporated with under reflux in vacuo. The 70% EtOH extract (398.5 g) was partitioned with n-hexane, CHCl3, EtOAc and n-BuOH. Partitions were then evaporated under a vacuum to afford the fractions of n-hexane (11.0 g), CHCl3 (17.7 g), EtOAc (8.3 g) and n-BuOH (117.0 g). The EtOAc fraction (8.3 g) was used for Medium Pressure Liquid Chromatography (MPLC; Buchi, Switzerland) and eluted with CHCl3: MeOH (100:0–0:100, gradient) to yield 7 subfractions (E1–E7). Subfraction E5 (80 mg, CHCl3: MeOH = 80:20) underwent prep-High-Performance Liquid Chromatography (HPLC; Waters, Singapore) and eluted with ACN: 0.1% acetic acid (10:90–100:0 for 60 min) to yield compound 2 (2 mg). The n-BuOH fraction (117.0 g) was used for MPLC and eluted with Water: MeOH (40:60–0:100, gradient) to yield 4 subfractions (B1–B4). Subfraction B1 (1.84 g, Water: MeOH = 40:60) underwent prep-HPLC and eluted with ACN: 0.1% acetic acid (10:90–55:45 for 30 min) to yield compound 1 (5 mg). Subfraction B3 (1.72 g, Water: MeOH = 20:80) underwent prep-HPLC and eluted with ACN: 0.1% acetic acid (20:80–75:25 for 30 min) to yield compounds 3 (3 mg).
Luteolin (1): Yellow powder; C15H10O6; EI-MS m/z: 286 [M]+. 1H-NMR (500 MHz, DMSO-d6): δ 7.40 (1H, dd, H-6’, J = 2.0, 8.5 Hz), 7.38 (1H, d, H-2’, J = 2.0 Hz), 6.86 (1H, d, H-5’, J = 8.5 Hz), 6.63 (1H, s, H-3), 6.41 (1H, d, H-8, J = 2.0 Hz), 6.15 (1H, d, H-6, J = 2.0 Hz); 13C-NMR (125 MHz, DMSO-d6): δ 181.5 (C-4), 164.9 (C-7), 163.8 (C-2), 161.4 (C-5), 157.3 (C-9), 150.2 (C-4’), 145.9 (C-3’), 121.1 (C-1’), 119.0 (C-6’), 115.9 (C-5’), 113.1 (C-2’), 103.4 (C-10), 102.6 (C-3), 99.0 (C-6), 93.9 (C-8).
Luteolin-7-O-β-D-glucoside (2): Yellow powder; C21H20O11; EI-MS m/z: 286 [M-sugar]+. 1H-NMR (500 MHz, DMSO-d6): δ 7.45 (1H, dd, H-6’, J = 2.5, 8.5 Hz), 7.42 (1H, d, H-2’, J = 2.5 Hz), 6.91 (1H, d, H-5’, J = 8.5 Hz), 6.79 (1H, d, H-8, J = 2.5 Hz), 6.76 (1H, s, H-3), 6.45 (1H, d, H-6, J = 2.0 Hz), 5.09 (1H, d, H-1’’, J = 7.5 Hz), 3.18–3.72 (sugar proton); 13C-NMR (125 MHz, DMSO-d6): δ 181.9 (C-4), 164.5 (C-2), 162.9 (C-7), 161.1 (C-5), 156.9 (C-9), 149.9 (C-4’), 145.8 (C-3’), 121.4 (C-1’), 119.2 (C-6’), 116.0 (C-5’), 113.6 (C-2’), 105.3 (C-10), 103.2 (C-3), 99.9 (C-1’’), 99.5 (C-6), 94.7 (C-8), 77.2 (C-3’’), 76.4 (C-5’’), 73.1 (C-2’’), 69.5 (C-4’’), 60.6 (C-6’’).
Luteolin-7-O-β-D-glucuronide (3): Yellow powder; C21H18O12; FABMS m/z: 417 [M-COOH]+. 1H-NMR (500 MHz, DMSO-d6): δ 7.45 (1H, d, H-6’, J = 2.0 Hz), 7.43 (1H, s, H-2’), 6.90 (1H, d, H-5’, J = 8.0 Hz), 6.79 (1H, d, H-8, J = 2.0 Hz), 6.75 (1H, s, H-3), 6.43 (1H, d, H-6, J = 2.0 Hz), 5.14 (1H, d, H-1’’, J = 7.5 Hz), 3.34–3.75 (sugar proton); 13C-NMR (125 MHz, DMSO-d6): δ 181.9 (C-4), 171.0 (COOH), 164.5 (C-2), 162.8 (C-7), 161.1 (C-5), 156.9 (C-9), 150.0 (C-4’), 145.8 (C-3’), 121.3 (C-1’), 119.1 (C-6’), 116.0 (C-5’), 113.5 (C-2’), 105.3 (C-10), 103.1 (C-3), 99.5 (C-1’’), 99.4 (C-6), 94.5 (C-8), 76.1 (C-3’’), 74.4 (C-5’’), 72.9 (C-2’’), 71.7 (C-4’’).
RAW 264.7 macrophages were gained from the American Type Culture Collection (ATCC, USA) and were cultured in DMEM supplemented with 10% FBS, penicillin (100 U/mL) and streptomycin (100 μg/mL), in a humidified incubator with 5% CO2 at 37°C.
The cell viability was measured using a cell counting kit-8 (CCK-8) assay (Dojindo Laboratoies, Japan). Cells were seeded at a density of 3 × 105 cells/mL into 96-well plates and incubated at 37°C in CO2 incubator for 24 h. Cells were then treatment with various concentrations (2.5–20 μg/mL) of compounds 1–3. CCK-8 was added after incubation for 24 h and plates were incubated for another 1 h at 37°C. The absorbance of each well was measured at 450 nm by a microplate reader (Molecular Devices, San Jose, CA, USA).
RAW 264.7 cells were cultured in 96-well plates (3 × 105 cells/mL) and incubated at 37°C for 24 h. Cells were then incubated with 5 μM DCFH-DA (2′,7′-Dichlorofluorescin diacetate) for 30 min in the dark, followed by treatment with various concentrations (2.5–20 μg/mL) of compound 1–3 and further incubation for 30 min. Cells were then exposed to 1 μg/mL LPS for 24 h. The ROS production was measured by recording fluorescence at an excitation/emission wavelength of 492/525 nm by fluorescence microplate reader.
Production of NO was determined by quantifying the amount of nitrite generated using the Griess reaction method (Chanput et al., 2016). Briefly, RAW 264.7 cells were plated in 24-well plates (3 × 105 cells/mL) and after reaching confluency, they were treatment with various concentrations of compounds 1–3 (2.5–20 μg/mL) and 1 μg/mL LPS for 24 h. Cultured medium was then collected and nitrite level was measured using the Griess reagent kit (Invitrogen, Carlsbad, CA, USA). Absorbance was measured at 548 nm by a microplate reader. Production of NO was expressed as a percentage (%) of the control value.
RAW 264.7 cells were plated in 24-well plates (3 × 105 cells/mL) and upon confluency, they were treatment with various concentrations of compound 1–3 (2.5–20 μg/mL) and LPS (1 μg/mL) for 24 h. PGE2 production was measured by PGE2 high sensitivity enzyme immunosorbent assay (ELISA) kit (Enzo, Tokyo, JAPAN). Absorbance was investigated at 405 nm using a microplate reader and PGE2 production was expressed as a percentage (%) of the control value.
Results
S. brachyotus was extracted with 70% EtOH, and MPLC and prep-HPLC were carried out, as a result of which three compounds (1–3) were isolated from EtOAc (compound 2) and n-BuOH (compound 1 and 3) fractions (Fig. 1, Table 1). All these compounds were isolated as a yellow powder. A characteristic doublet signal of 5,7-dihydroxy flavone exhibited at δ 6.15 of H-6 and δ 6.41 of H-8 in the 1H-NMR spectrum of 1, and a doublet signal of 7-O-glycosides of 5,7-dihydroxy flavone exhibited at δ 6.43-6.45 of H-6 and δ 6.79 of H-8 in the 1H-NMR spectra of 2 and 3 were observed. The downfield shift of H-6 and H-8 of 2 and 3 was indicative of a substitution by sugar moiety of C-7. Further, a typical ABX system signal showed a doublet at δ 7.38–7.42 of H-2’, a doublet at δ 6.86–6.91 of H-5’, and a double doublet at δ 7.40–7.45 of H-6’ of 1–3, respectively. The 13C-NMR spectra of all four compounds showed similar structural signals of 15 carbons which were related to flavone structure. Compounds 1–3 were distinguished by the existence and nonexistence of sugar in the A-ring. Compound 1 was aglycone of compounds 2–3 and was elucidated as luteolin by comparison of its EI-MS and NMR (1D- and 2D-NMR) spectral data with those previously published in the literature (Lee et al., 2011). The anomeric signals of compound 2 and 3 were observed at δ 5.08–5.14 and δ 99.5–99.9 in 1H-NMR and 13C-NMR spectra, respectively. In the HMBC experiment, correlations between δ 5.08–5.14 (anomeric proton) and δ 162.8–162.9 (C-7) confirmed that anomeric proton was attached to C-7 of A-ring in compound 2 and 3. Compound 2 was identified by a carbon signal (δ 60.6–99.9) of typical glucose and was elucidated as luteolin-7-O-β-D-glucoside by comparison of its spectral data with those of a previously published study (Lee et al., 2011). Compound 3 showed a signal that was assigned to the carboxyl group (δ 171.0) of glucuronic acid, and it was elucidated as luteolin-7-O-β-D-glucuronide by comparison of its spectral data with those described in a previous study (Ozgen et al., 2010).

We evaluated cytotoxicity of compounds 1–3 using CCK-8 assay prior to investigating their inhibitory effect on the generation of intracellular ROS. Compounds 1–3 didn’t affect the viability of LPS-stimulated RAW 264.7 cells (2.5–20 μg/mL) (Fig. 2A). Therefore, concentration range of 2.5–20 μg/mL was selected for subsequent experiments. To examine the inhibition of ROS production by compounds 1–3, we measured ROS levels using DCFH-DA reagent. ROS generation was increased significantly in LPS-exposed cells; however, it was inhibited effectively by all four compounds in a concentration-dependent manner (Fig. 2B). In particular, compound 1 at 20 μg/mL inhibited the production of ROS by 48.6% compared to the other compounds.
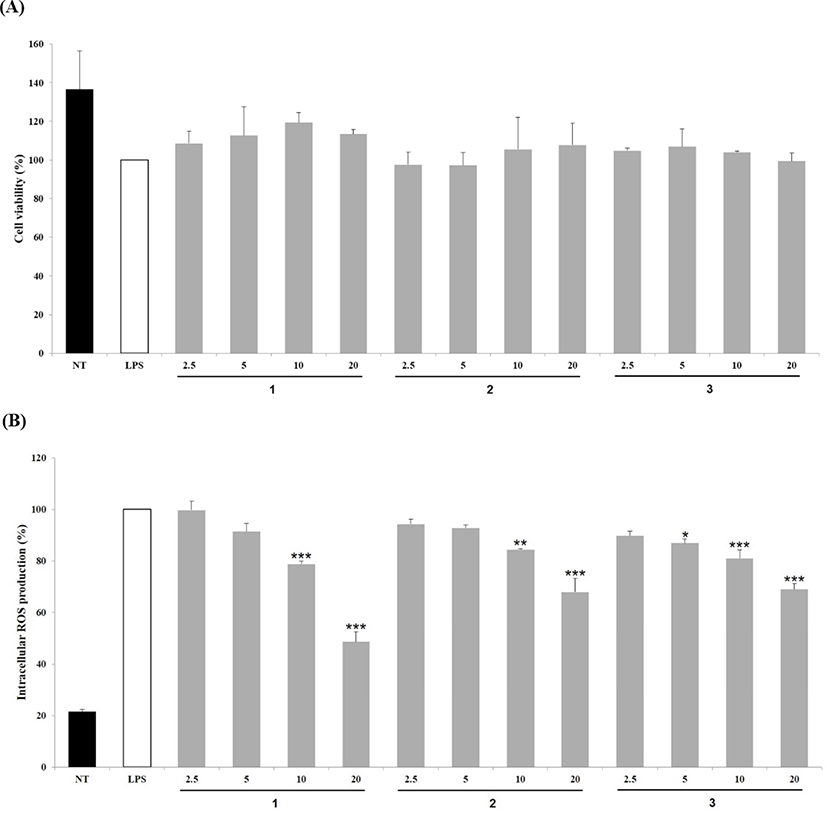
Compounds 1–3 (2.5–20 μg/mL) were evaluated inhibitory effects on NO production in LPS-stimulated RAW 264.7 cells. As shown in Fig. 3A, NO production was increased significantly in cells exposed to LPS, whereas treatment with all four compounds at 20 μg/mL suppressed NO production effectively. Particularly, in cells treated with compounds 1 and 2 (20 μg/mL), NO production was inhibited by 61.28 and 67.03%, respectively.
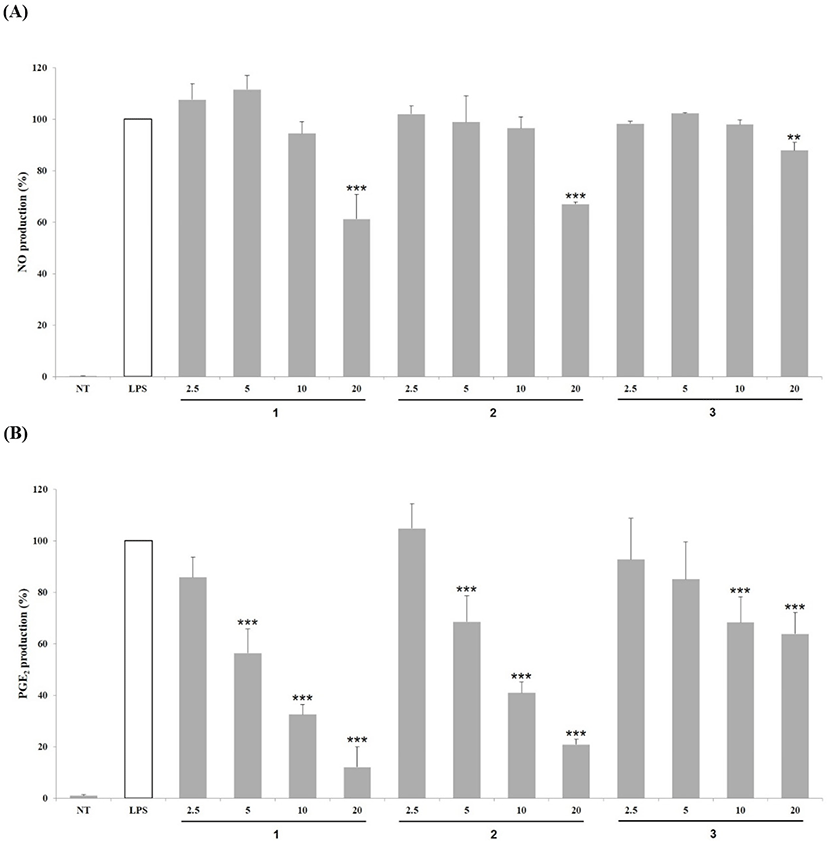
Inhibitory effects of compounds 1–3 (2.5–20 μg/mL) on PGE2 production was evaluated in LPS-stimulated RAW 264.7 cells. As shown in Fig. 3B, compounds 1–3 decreased PGE2 production in a concentration-dependent manner. Compounds 1 and 2 at 20 μg/mL suppressed PGE2 production by 12.10% and 20.82%, respectively, which is indicative of their stronger inhibitory effect than that of the other two compounds.
Discussions
S. brachyotus is a perennial plant belonging to Asteraceae family and its young leaves have been used for medicinal resources in Korea (Nugroho et al., 2012). S. brachyotus has been shown to exhibit various biological activities, such as antioxidant, anti-inflammatory, antibacterial and antidiabetic activities (Lee et al., 2018; Li et al., 2017; Xia et al., 2011); however, very limited knowledge is available regarding the isolation and identification of its secondary metabolites. Therefore, in the current study, we isolated secondary metabolites from S. brachyotus and evaluated their antioxidant and anti-inflammatory activities. Isolation of these secondary metabolites was carried out using a 70% EtOH extract of S. brachyotus, and they were identified as luteolin (1), luteolin-7-O-β-D-glucose (2), and luteolin-7-O-β-D-glucuronide (3). In earlier studies, luteolin (1), luteolin-7-O-β-D-glucose (2), and luteolin-7-O-β-D-glucuronide (3) have been isolated from Sonchus species, such as S. oleraceus, S. erzincanicus (Ozgen et al., 2010; Yin et al., 2008).
To investigate biological activities (antioxidant and anti-inflammatory) of the three isolated flavonoids, we evaluated inhibitory effect on the production of ROS, NO and PGE2. As a result of our experiments, all the three compounds inhibited ROS generation which is indicative of their antioxidant activity, however, luteolin (1) at 20 μg/mL showed the strongest antioxidant activity (Fig. 2B). Among three compounds, luteolin (1) and luteolin-7-O-β-D-glucose (2) at 20 μg/mL showed similar inhibitory effects on the production and secretion of inflammatory mediator NO and PGE2. Luteolin (1) and luteolin-7-O-β-D-glucose (2) are common flavonoids found in plants and have been previously reported to show antioxidant and anti-inflammatory activities. Antioxidant activity of these compounds was demonstrated by determining their inhibitory activity against lipid peroxides formation and ROS generation, DPPH and ABTS radical scavenging activity, oxygen-radical absorbance capacity, and their ability to inhibit xanthine oxidase activity (Chanput et al., 2016; Lee et al., 2016; Özgen et al., 2011). The anti-inflammatory activity of luteolin (1) and luteolin-7-O-β-D-glucose (2) was evaluated in LPS-stimulated THP-1 monocytes, and by determining their inhibitory effect against the production of NO and PGE2 secretion (Chanput et al., 2016; Lee et al., 2015; Lee et al., 2016; Park & Song, 2013). In agreement with previous reports, we observed that luteolin (1) and luteolin-7-O-β-D-glucose (2) isolated from S. brachyotus exerted strong antioxidant and anti-inflammatory activities. In addition, luteolin (1) and luteolin-7-O-β-D-glucose (2) have been shown to exhibit various biological activities such as antimicrobial (Lee et al., 2010), anti-cancer (Yamabe et al., 2014), and anti-diabetic effects (Zang et al., 2016).
Conclusion
We successfully isolated and identified three flavonoids namely luteolin (1), luteolin-7-O-β-D-glucoside (2), and luteolin-7-O-β-D-glucuronide (3) from S. brachyotus 70% EtOH extract. S. brachyotus extract containing luteolin (1) and luteolin-7-O-β-D-glucose (2) is considered to exert diverse biological activities apart from antioxidant and anti-inflammatory activities, which were investigated in this study. Thus, S. brachyotus, which is a common edible medicinal plant, can be regarded as an important source of functional foods.