Introduction
Fish meal (FM) replacement with alternative protein sources is considered a major research area in aqua feed industry over the last few decades due to limited FM supply and higher demand. Fisheries by-products and other marine protein sources have been used in FM replacement studies as supplements to compensate impaired growth performance caused by FM reduced diets (Khosravi et al., 2015a; Leal et al., 2010; Leduc et al., 2018; Plascencia-Jatomea et al., 2002). As the result, advanced processing technologies have been developed to improve the quality of the by-products (Chalamaiah et al., 2012; Dong et al., 2008).
Shrimp hydrolysates (SH) are produced from shrimp by-product or processing wastes such as shrimp heads, cuticles or tail muscles. In particular, shrimp cephalothorax and exoskeleton contain asthaxanthin and protein those are account for 35%–45% of whole-body weight as inedible wastes (Meyers, 1986; Shahidi and Synowiecki, 1991). Therefore, several methods were exploited to make the by-products edible or valuable. Hydrolysis method was recommended as a successful way to convert by-products into micro nutrients (Bueno-Solano et al., 2009; Cahú et al., 2012; López-Cervantes et al., 2006; Nwanna, 2003). The studies on SH have revealed antioxidant, antihypertensive, antimicrobial and myotropic activities (Huang et al., 2011; Kleekayai et al., 2015; Leduc et al., 2018; Nii et al., 2008). Trace amounts of heavy metals, such as Hg, Pb and Cd were recorded in shrimp by-products while free amino acid (AA) content was 15% higher than the edible parts of shrimp (Heu et al., 2003). In this regard, SH has been evaluated in diets for fish and shrimp species, and positive effects were elucidated on growth, immunity, digestibility, palatability and health (Khosravi et al., 2015a, 2015b; Khosravi et al., 2018; Leal et al., 2010; Leduc et al., 2018; Plascencia-Jatomea et al., 2002).
Krill are small crustaceans classified into order Euphausiacea. They are one of the primary food sources for large marine mammals and fishes (Hardy, 2008) and a rich source of marine proteins, omega 3 fatty acids, phospholipids and astaxanthine. Therefore, krill processing by-products are used to produce valuable products, such as krill meal (KM), krill hydrolysates and krill oil (Tilseth & Høstmark 2014; Xie et al., 2019). KM is a palatability enhancer in addition to suitable lipid and mineral source for fish (Goto et al., 2001; Hansen et al., 2010). Therefore, increased feed intake (FI) has been reported in fish species when diets are incorporated with KM (Hatlen et al., 2017; Yoshitomi et al., 2006). Dietary KM supplementation have been proven to improve growth, feed utilization, health status, diet digestibility and disease resistance of fish (Hansen et al., 2010; Yan et al., 2018). Recently, FM replacement ability of KM was reported by several studies in diets for different fish species including gilthead seabream (Sparus aurata) (Saleh et al., 2018), large yellow croaker (Larimichthys crocea) (Wei et al., 2019), and olive flounder (Paralichthys olivaceus) (Tharaka et al., 2020). KM was also included as a feed ingredient in FM reduced diets for red seabream (Pagrus major) (Takagi et al., 2001; Kader et al., 2012). Shimizu at al. (1990) reported that feeding behavior of red seabreams was stimulated by dietary KM supplementation. Later, Kader et al. (2010) replaced 60% of FM protein with soy protein concentrate (SPC) and KM in red seabream diet without compromising feeding behavior, growth or health. Recently, Cho et al. (2018) tested KM as a main protein source in red seabream diet and observed that AA and fatty acid profiles of the fish were improved by KM.
Red seabream is cultured within East Asian region. It was the second largest cultured fish species in Japan (Koshio, 2002) and the third one in Korea (Kim et al., 2012; KOSTAT, 2017). In our previous studies, FM replacement was successful with SH and krill hydrolysates from a high-FM (HFM) diet for red seabream (Bui et al., 2014) and HFM effects were restored in diets containing high proportion of plant protein (Khosravi et al., 2015a). Therefore, this study was conducted to examine the effects of SH or KM in a high-plant-protein diet for red seabream compared to a low-FM (LFM) and HFM diets and, meanwhile, compare the supplemental effects of SH and KM in LFM diets for red seabream.
Materials and Methods
Four diets were formulated to contain 45% crude protein and 18 kJ/g energy (Table 1) by inclusion of SH and KM those were provided by DIANA AQUA (Aquaculture Division of DIANA, Member of SYMRISE Group, Elven, France). The molecular weight distribution, proximate and essential AAs composition of SH and KM were presented in Table 2. A diet prepared to contain 40% FM was considered as a HFM diet. Another diet was prepared to contain 25% FM and 21% SPC, was regarded as the LFM diet. Two other experimental diets (SH and KM) were prepared by including SH or KM into the LFM diet at 5% inclusion levels in exchange of 5% FM. Both SH and KM diets contained 50% of FM compared to the HFM diet. All dry ingredients were thoroughly mixed and, after addition of oil and distilled water, the dough was extruded through a pelletizer machine (SP-50, Gum Gang Engineering, Daegu, Korea) in ideal size dried at 25°C for 24 h and stored at −20°C.
4) Mineral premix (g/kg of mixture): MgSO4 · 7H2O, 80.0; NaH2PO4 · 2H2O, 370.0; KCl, 130.0; Ferric citrate, 40.0; ZnSO4 · 7H2O, 20.0; Ca-lactate, 356.5; CuCl, 0.2; AlCl3 · 6H2O, 0.15; Na2Se2O3, 0.01; MnSO4 · H2O, 2.0; CoCl2 · 6H2O, 1.0.
5) Vitamin premix (g/kg of mixture): L-ascorbic acid, 121.2; DL-α tocopheryl acetate, 18.8; thiamin hydrochloride, 2.7; riboflavin, 9.1; pyridoxine hydrochloride, 1.8; niacin, 36.4; Ca-D-pantothenate, 12.7; myo-inositol, 181.8; D-biotin, 0.27; folic acid, 0.68; p-aminobezoic acid, 18.2; menadione, 1.8; retinyl acetate, 0.73; cholecalficerol, 0.003; cyanocobalamin, 0.003.
The feeding trial was conducted in the Marine Science Institute, Jeju National University, Jeju, Korea. All fish were acclimatized for two weeks by feeding a commercial diet. Fish (8.47 ± 0.05 g) were randomly selected and distributed among twelve polyvinyl circular tanks (30 fish / tank) of a flow-through system in which water flow tare was maintained at 3 L/min and air stones were installed to provide oxygen. Tanks were randomly assigned to three replicates of four dietary treatments. Photoperiod was scheduled for 12:12 h light/dark by fluorescent light. Water temperature was ranged from 22.6°C to 25.3°C during the feeding trial. Ammonia level and pH were around 0.04 ppm and 7.3 respectively. Fish in each tank were fed one of the experimental diets twice a day (09:00 and 18:00 h) until satiation for fifteen weeks. Fish growth was measured in three weeks interval. Fish were fasted for 24 h prior to weighting to minimize stress. FI was calculated by collecting uneaten feed 30 min after feeding.
All the fish from each tank were counted to determine survival rate and bulk weighed to determine growth performance and feed utilization after fifteen weeks of feeding trial. Then, six fish were randomly selected from each tank and anesthetized with 2-phenoxy ethanol solution (200 ppm) by dipping for few minutes. Three fish were selected to withdraw blood samples with syringes containing heparin to separate plasma samples. Another three fish were selected to withdraw blood samples with non-hepasrinized syringes and the serum was separated after clotting at room temperature. Both plasma and serum were separated after centrifugation at 5,000 × g for 10 min and stored at −80°C for further analyses. Three fish were sampled from each tank to analyze whole-body proximate composition.
Standard analytical procedures (AOAC, 2005) were followed to analyze moisture and ash contents. Crude protein was determined by using a Kjeltec Analyzer Unit 2300 (FOSS analytical, Hilleroed, Denmark). Protocol of Folch et al. (1957) was followed to determine crude lipid levels. Hematocrit was measured by microhematocrit technique (Brown, 1980). Plasma biochemical parameters were determined using a blood analyzer (SLIM, SEAC, Florence, Italy). Nitroblue tetrazolium (NBT) assay was performed to determine respiratory burst activity as described by Anderson and Siwicki (1995). Myeloperoxidase (MPO) activity of serum was measured according to Quade and Roth (1997). Serum superoxide dismutase (SOD) activity was measured using a Kit (19160, Sigma-Aldrich, St. Louis, MO, USA). Catalase and glutathione peroxidase (GPx) activities of serum samples were measured using commercial kits (K773-100 and K762-100, Biovision, Milpitas, CA, USA).
Apparent digestibility coefficients (ADCs) of protein and dry matter (DM) of the diets were determined using a Guelph system. For ADCs, the experimental diets were added with 1% chromic oxide (Cr2O3) (203068, Sigma-Aldrich) which is an inert indicator. Four groups of red seabream (average body weight: 60 g) were distributed into tanks (300 L capacity) at a density of 100 fish per tank and fed with the diets containing chromic oxide. The Guelph system was settled to receive cartridge-filtered seawater at 2 L/min flow rate. Fecal samples were collected for 2 weeks. Chromic oxide levels were determined according to Divakaran et al. (2002). ADCs for protein and DM of the diets were calculated according to Tharaka et al. (2020).
Three fish were selected from each tank and livers were separated. Then, samples were immediately frozen in liquid nitrogen. RNA was isolated using TRIzol Reagent (Sigma-Aldrich) and concentrations were checked at 260 nm using a spectrophotometer after diluting in 0.1 mL of RNase free water. After, total RNA (2.5 μg) were reverse transcribed to cDNA with a kit (6110A, TaKaRa, Shiga, Japan) and volume up to 0.8 mL with RNase free water. The expression level of IGF-I gene was analyzed with a Real Time System (TP800 Thermal Cycler Dice™, TaKaRa, Shiga, Japan) by using a fluorescent agent (TB Green). The 18S rRNA gene was used as the housekeeping gene and primers for 18S rRNA gene were designed following a cloned sequence (NCBI Genbank accession no: AB259837). Previously designed primers were used for IGF-I gene (Hossain et al., 2016). The relative expression ratio of the gene was calculated following the described model by Pfaffl (2001): Ratio = [(EIGF-I) ΔGF (control-sample)] / [(Eactin) Ct (control-sample)].
Experimental diets were assigned using a completely randomized design. The differences among groups were identified by one-way analysis of variance (ANOVA) in SPSS version 19.0 (SPSS, Chicago, IL, USA). Then, Tukey’s HSD test was used to find differences in mean values (p < 0.05). The percentage data were arcsine transformed before the comparisons. All the data are presented as mean of three replicates ± SD.
Results
All diets were well accepted and consumed by the fish during the feeding trial. Final body weight, weight gain, specific growth rate, feed conversion ratio (FCR) and protein efficiency ratio (PER) were significantly improved in fish fed diets containing HFM, KM, or SH compared to those of fish fed LFM diet. Interestingly, the parameters were significantly higher in fish fed SH diet than those of fish fed KM and HFM diets. FI and survival of fish were not significantly affected by the dietary treatments. Liver IGF-I mRNA expression of fish fed SH diet was in line with HFM group and significantly higher compared to that of fish fed KM and LFM diets. KM group showed significantly higher expression level compared to LFM group. The lowest expression level was observed in fish fed LFM diet (Table 3).
DM and protein digestibility of diets were significantly enhanced by inclusion of SH and KM (Fig. 1). Protein digestibility of SH diet was significantly higher than KM, HFM, and LFM diets. Protein digestibility of KM and HFM diets were significantly higher than LFM diet. DM digestibility of SH diet was significantly higher than KM and LFM diets although values observed in SH and KM diets were comparable to HFM diet. The lowest protein and DM digestibility were found in LFM diet.
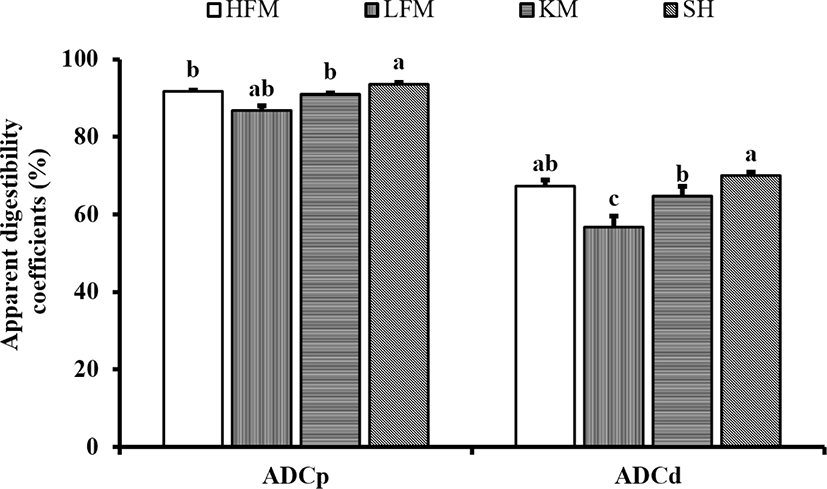
The results of innate immune and antioxidant enzyme analyses are shown in Table 4. Innate immune responses of fish fed SH diet were significantly enhanced compared to those of fish fed the LFM diet. Even though SH group showed the most improved innate immunity, NBT and SOD activities of HFM and KM groups were significantly elevated than the LFM group. However, catalase and GPx activities of KM group were comparable to LFM group. MPO activity was not significantly affected by diets.
The results of blood parameters are shown in Table 4. Hemoglobin level and hematocrit of fish fed SH and KM diets were significantly higher than that of fish fed LFM diet. However, cholesterol, glucose and total protein levels of fish were not significantly affected by the diets. No significant differences were observed in whole-body composition of fish (Table 5).
Discussion
A LFM diet containing SH improved fish growth and exerted even better performance than the HFM group. Also, growth performance of fish fed KM diet was comparable to HFM group. In our previous studies, growth performance of red seabream was improved by supplementation of 5% SH compared to a HFM diet (Bui et al., 2014). HFM effects were shown by red seabream fed a SPC based LFM diet containing SH (Khosravi et al., 2015a). FI of fish was not significantly influenced by the experimental diets in the present study. Therefore, increased fish growth by SH or KM supplementation might be due to the nutritional quality of diets which was represented by improved FCR and PER compared to the LFM group. KM and SH have balanced essential AAs and fatty acids for fishes. Soluble protein level is high in SH which contains high level of low molecular weight peptides (Table 2). Many active peptides, known as growth promoter in fish, are found in these molecular ranges (Chalamaiah et al., 2012). Also, crude lipid level and omega-3 fatty acids and EPA , are high in KM. SH and KM contain astaxanthin which is a growth stimulator of fish (Cheng et al., 2018; Kalinowski et al., 2011; Li et al., 2014; Li et al., 2018; Lim et al., 2018; Xie et al., 2017). Therefore, these compounds might be involved in restoring HFM effects by SH or KM supplementation into the LFM diet. Teshima et al. (2004) reported that dietary supplementation of low molecular weight peptides enhances the growth performance of red seabream. Kondo et al. (2017) demonstrated that feed conversion and expression of digestive enzymes of red seabream can be improved by dietary protein hydrolysate supplementation. Supportively, low molecular compounds and peptides which are generated during the process of hydrolysis might be associated with the enhanced growth performance of fish fed diets containing SH compared to the KM group.
IGF-I is identified as a mitogenic polypeptide that can mediate somatic growth-stimulatory effects of growth hormone. It is secreted by the liver and transported to other tissues in vertebrates (Laron, 2001; Merimee & Laron, 1996). In the same way, IGF-I in teleost is regulated by growth hormone and secretion of hepatic IGF-I in a dose-dependent manner (Shamblott et al., 1995). IGF-I plays an important role to develop nervous system and muscle during the larval stage of Chilean flounder (Paralichthys adspersus) (Escobar, 2011). Furthermore, McCormick (1996) demonstrated that this gene might be involved in osmoregulation of Atlantic salmon (Salmo salar). In the present study, relative expression levels of liver IGF-I mRNA of LFM fish group did not reach to those of HFM fish group. However, KM supplementation resulted in upregulated expression level of the LFM group and dietary SH supplementation restored the HFM effects. Bjørndal et al. (2012) observed that dietary krill powder can upregulate the expression of animal genes. Zheng et al. (2012) found that liver IGF-I expression of Japanese flounder (Paralichthys olivaceus) was significantly increased by replacing 16% FM with protein hydrolysates. The present results along with the previous ones indicate that the SH, as a hydrolysate, could recover a decreased IGF-I expression of fish fed a diet containing high-plant protein sources even though the exact mechanism was not clearly verified. According to Metón et al. (2000), IGF-I expression of gilthead seabream can be changed after feeding. They observed normalized expression about 16 h after the last feeding. Therefore, IGF-I expression might not be changed with sample collection time in the present study because fish were fasted for 24 h before sampling. Plasma IGF-I level was also found to have a correlation with the protein contents of isocaloric diets (Moriyama et al., 2000). SH and KM used for this study contain almost similar level of AAs and proteins. Whereas, soluble protein level in SH was higher compared to KM (Table 2). Therefore, high levels of soluble protein in SH might explain the reason for the increased IGF-I expression.
In our previous studies, SH supplementation improved protein and DM digestibility of red seabream compared to a HFM diet (Bui et al., 2014). Digestibility of red seabream diet is dependent on several factors such as feeding rate (Takii et al., 1997), photoperiod (Biswas et al., 2005) and stocking density (Biswas et al., 2007). Dossou et al. (2018) reported that FM could be substituted up to 25% with fermented rapeseed meal without compromising digestibility of protein in red seabream diet. However, LFM diet showed significantly lower protein digestibility in the present study indicating that SPC was not capable to replace FM in red seabream diet down to 25% without compromising protein digestibility. SH and KM groups restored the HFM effects by increasing diet digestibility. Kader and Koshio (2012) improved diet digestibility by incorporating 20% of FM with fermented soybean meal and fish soluble in red seabream diet. Protein and AA digestibility of red seabream vary with protein sources in diet (Yamamoto et al., 1998). Therefore, in line with these findings, it can be assumed that proper level of SH was included in a LFM diet for red seabream possessing an ability to enhance protein and DM digestibility of the diet. KM supplementation was reported increased digestibility of no-FM diet for Nile tilapia (Oreochromis niloticus) (Gaber, 2005). Tibbetts et al. (2006) observed that protein digestibility of Atlantic cod (Gadus morhua) was improved by KM supplementation. However, in our study, protein and DM digestibility were higher in SH diet than that of KM diet. Nutritional quality of SH, especially higher levels of low molecular weight compounds, seems to be the main reason for the improved protein digestibility of diet compared to KM or HFM diets.
Innate immune system is considered as the core defense mechanism found in fish for combating infections (Magnadóttir, 2006). In the present study, NBT activity and antioxidant enzymes such as serum catalase, SOD and GPx activities were significantly enhanced by dietary inclusion of SH or KM in a LFM diet. SH supplementation resulted in even higher performance than HFM group. According to our previous studies, innate immunity of red seabream was boosted by SH incorporation into a LFM diet restoring the positive HFM effects (Khosravi et al., 2015a). Innate immune system of olive flounder was also stimulated by dietary SH (Gunathilaka et al., 2020; Khoshravi et al., 2018) and KM (Tharaka et al., 2020) supplementation. Innate immunity of Japanese sea bass (Lateolabrax japonicas) and Atlantic halibut (Hippoglossus hippoglossus) was enhanced by dietary supplementation of hydrolysates from Pollock by-product (Hermannsdottir et al., 2009; Liang et al., 2006). Siddik et al. (2019) reported that immune responses of Asian seabass (Lates calcarifer) can be improved by tuna hydrolysates supplementation in diets. Immunostimulatory effects of different hydrolysates products have been revealed over past decade (Chalamaiah et al., 2015; Chalamaiah et al., 2018; Huang et al., 2014; Karnjanapratum et al., 2016). Dietary AAs were reported to boost immunity and antioxidant enzyme activities of fishes (Machado et al., 2015; Pohlenz et al., 2012). Both SH and KM contain high levels of AAs although the SH contained high soluble nitrogen level and low-molecular compounds than KM. Therefore, SH might contain nucleotides and peptides among low molecular weight compounds. Both nucleotides and peptides were identified as immune modulators in animals including fishes (Cederlund et al., 2011; Chiou et al., 2006; de Cruz et al., 2020; Harris and Bird, 2000; Rajanbabu and Chen, 2011; Valero et al., 2020). Therefore, innate immunity of red seabream might be boosted more efficiently by dietary SH supplementation compared to KM which was not hydrolyzed. Evidences from previous studies elucidated that chitin present in KM have ability to improve immunity of fish (Ringø et al., 2012). Several studies reported that chitin improve disease resistance of fish along with the immunity (Ringø et al., 2012). Improved immunity is properly reflected by a disease challenge against virulent pathogen in red seabream aquaculture. Therefore, we suggest to conduct immune based challenge test in future experiments to illustrate immune boosting effects of LFM diets containing SH and KM.
Blood hematocrit and hemoglobin level of fish significantly improved by dietary KM and SH supplementation compared to LFM group. Olsen et al. (2006) reported that dietary KM supplementation had no adverse effects on hematocrit, hemoglobin or red blood cell count of Atlantic salmon. Also, Silva-Carrillo et al. (2012) replaced FM from juvenile spotted rose snapper (Lutjanus guttatus) diet with soybean meal up to 60% without adverse effects on hematocrit and hemoglobin level when diets were supplemented with KM and Squid meal. Khosravi et al. (2015a) observed increment in blood hemoglobin and hematocrit by dietary SH supplementation. High level of plant protein in diets causes to reduce hemoglobin level and hematocrit of fish (Haghbayan et al., 2015). Accordingly, in our study, blood hemoglobin level and hematocrit were reduced by LFM diet due to high level of SPC and increased by KM and SH to reach HFM effects. The improved nutritional status as represented by growth performance, feed utilization and diet digestibility of SH and KM group might be connected with restored hemoglobin and hematocrit levels.
Conclusion
The results observed by this study provide evidences to prove that the 20% of FM inclusion in red seabream diet with KM is comparable to a HFM diet which contain 40% FM. Also, compared to KM, SH can be used to replace FM from red seabream diet down to 20% and fish performance can be maintained better than a diet containing 40% FM. Therefore, inclusion of SH in LFM diet is more effective than KM to improve growth, digestibility and immunity of red seabream.