Introduction
In recent years, viruses have become a field of interest to public health due to the emergence of zoonotic diseases. Viruses can cross species barriers into other host populations, including humans, and approximately 70% of the emerging pathogens infecting humans originated from animals (Chan et al., 2013). For example, bats are presumed to be reservoirs of diverse coronaviruses, including the causative agents of severe acute respiratory syndromes (SARS) and coronavirus disease-19 (COVID-19) in humans (Forni et al., 2017; Latinne et al., 2020; Tao et al., 2017). It is also well known that avian influenza viruses occasionally transmit zoonotically to humans (Malik Peiris, 2009). In addition, swine influenza viruses are readily transmitted to humans by contact with respiratory secretions from infected pigs as well as the air (Van Reeth, 2007). Moreover, the influenza A virus has been reported in both pinnipeds and cetaceans, and 4 people developed conjunctivitis after the post-mortem examination of H7N7-infected seals from an outbreak in New England (Geraci et al., 1982; Webster et al., 1981). Many of the emerging diseases that threaten humans are caused by RNA viruses and this is not by chance as RNA viruses are extremely mutable and use very efficient strategies for generating viral diversity during evolution (Webby et al., 2004). Therefore, animal RNA viruses are most, although not exclusively, able to achieve successful adaptations to human hosts (Webby et al., 2004).
To date, viral transmission from aquatic animals to humans appears to be very rare. Calicivirus is perhaps the only real case of transmission from fish (opaleye; Girella nogricans) to several species of mammals including sea lions, fur seals, swine, and humans (Smith & Boyt, 1990). People infected with this RNA virus showed mild to severe vesicular lesions on the hands and feet (Smith et al., 1998). Scientists have investigated the possibility of interspecies transmission between fish and mammals at the in vitro level. A few fish viruses showed the ability to infect non-piscine cell lines at the temperatures commonly used to propagate these viruses. For example, spring viremia of carp virus, infectious hematopoietic necrosis virus, and viral hemorrhagic septicemia virus (VHSV) were all able to replicate efficiently and cause cytopathic effects (CPEs) in lizards (gecko lung-1), hamsters (BHK/21), and humans (WI-38) at 23°C, 18°C, and 18°C, respectively (Clark & Soriano, 1974). In addition, the striped bass (SBR) virus was able to replicate in monkey (Vero) and bovine cell lines at 22°C with a viral titer similar to that in fish cells. Similarly, Lorenzen & Olesen (1995) showed that VHSV could replicate in cabbage looper cells (Trichoplusia ni) at 15°C with a viral titer similar to that in fish cell lines, and the virus grown in an insect cell line was pathogenic to rainbow trout (Oncorhynchus mykiss). Although these results imply that those fish RNA viruses might infect humans, there is no clear information on the possible transmission from fish to humans at the in vivo level.
The known host range for VHSV has expanded enormously, including the families of Salmonidae, Esocidae, Clupeidae, Gadidae, and Pleuronectidae, as this RNA virus can cross species barriers (Crane & Hyatt, 2011; Schönherz et al., 2013). Hence, VHSV has become the most important pathogen of fish including species that are popularly consumed by humans worldwide. A previous study (Pham et al., 2011) demonstrated that VHSV could survive on the surface of a 96-well cell culture plate for 6 days at 37°C. However, the survival of VHSV varied depending upon isolates as the survival of some VHSV isolates inoculated into epithelioma papulosum cyprinid (EPC) cells at 20°C was significantly reduced, whereas some VHSV isolates replicated well in the same conditions (Arkush et al., 2006). Olesen & Jørgensen (1992) found that VHSV titers in the BF-2 cell line at 15°C were 2 times higher than those in EPC and CHSE-214 cells, indicating that the replication of VHSV varied depending upon the cell line. Clark & Soriano (1974) showed that VHSV could replicate and cause CPEs in mouse- and human-derived cells at 18°C. This was only one in vitro study using a human cell line, and there were no attempts to determine the infectivity of VHSV using mammalian model organisms. Therefore, this study aimed to determine the possible harmful effect of VHSV in humans using human and mouse cell lines at different temperatures as well as a challenge test in a mouse model.
Materials and Methods
Three cell lines were used in this study. SNU-1411 and hepa-1c1c7s cells derived from human rectum and mouse liver, respectively, were purchased from the Korean Cell Line Bank (KCLB) and EPC cells were purchased from the American Type Culture Collection (ATCC no. CRL-2872). For cell maintenance, the SNU-1411, hepa-1c1c7s, and EPC cell lines were cultured in RPMI1640 medium (SNU-1411), minimum essential media (MEM; hepa-1c1c7s and EPC cell lines) supplemented with 150 IU · mL–1 penicillin and 100 μg · mL–1 streptomycin (P/S, Gibco, Grand Island, NY, USA) and 10% fetal bovine serum (FBS, Gibco) (referred to RPMI1640-10 and MEM-10).
Virus stock was prepared according to a previous study (Ho et al., 2020). Briefly, VHSV was inoculated into EPC monolayers in MEM medium supplemented with 150 IU · mL–1 penicillin and 100 μg · mL–1 streptomycin (P/S) and 2% FBS (MEM-2) in 75 mL culture flasks. Seven days after incubation at 15°C, CPEs were examined. The supernatant containing VHSV was collected by centrifuging at 4,000×g for 10 min at 4°C and filtered using a syringe filter (0.45 μm). The supernatant containing the virus was stored at –80°C until use. The concentration of the viral stock solution was determined using the 50% tissue culture infective dose (TCID50) method (Reed & Muench, 1938) with some modifications. Briefly, one hundred microliters of EPC cells (1E+06 cell · mL–1) were seeded into 96-well plates. Viral stock solution (100 µL) at different 10-fold dilution levels was inoculated into 5 wells. The plates were incubated at 15°C and observed for CPEs for 7 days. The number of wells that produced CPEs were counted and calculated to determine the TCID50 value.
The viral stock solution was diluted in MEM-2 to 4.22E+06 TCID50 · mL–1. The diluted stock (20 mL) was put into 6 T25 cell culture flasks with a closed cap. Three flasks were incubated at 15°C as controls, and the other 3 were incubated at 37°C. At 0, 1, 4, and 8 days post-inoculation (dpi), the culture medium (1 mL) was taken to determine the viral titer using the TCID50 method.
This experiment was done at the same temperatures as the previous one in mammalian cell lines including SNU-1411 and hepa-1c1c7s cell lines. The experiment at 37°C was performed independently of that at 15°C, resulting in different viral initial concentrations (7.94E+04 TCID50 · mL–1 and 4.22E+06 TCID50 · mL–1, respectively). Viral dilutions (20 mL) were inoculated into flasks containing monolayers of SNU-1411 and hepa-1c1c7s cells. A similar volume of RPMI1460 and MEM medium supplemented with 2% FBS was also added to the SNU-1411 and hepa-1c1c7s cell lines, respectively, as the negative control groups. Flasks were observed for CPEs at 0 and 16 dpi under a microscope. At 0, 1, 4, 8, 12, and 16 dpi, the culture supernatant (1 mL) was collected to determine the viral titer outside the host cells using the TCID50 method. The remaining culture supernatant was discarded and the cell layer was washed 3 times with phosphate-buffered saline (Sigma-Aldrich, Steinheim, Germany) to remove the unbound viruses. Subsequently, flasks were treated with 5 mL of trypsin (Gibco) to detach the cells. The treated SNU-1411 and hepa-1c1c7s cells were suspended in RPMI1460 or MEM medium (15 mL), respectively. The suspended cells (1 mL) were collected and centrifuged at 4,000×g for 10 min at 4°C. The supernatant was discarded and the cell pellet was suspended in 200 µL of the corresponding medium. The pellet was then used to determine the invaded virus.
Healthy mice (Mus musculus, 27.3 ± 1.9 g) were obtained from Central Lab. Animal (Seoul, Korea) and acclimated for 3 days. During acclimation, the mice were fed commercial feed. One day before starting the experiment, mice feeding was stopped. The experiment was performed in the animal laboratory of Gyeongsang National University, Korea (approval number: GNU-190808-M0036). There were 2 groups in triplicate including the control and VHSV-injected groups. Each group contained 5 mice. The mice were anesthetized by intraperitoneal injection of 500 µL of anesthetic medium (39.5 mL of 0.9% sodium chloride and 0.5 mL of avertin stock (25 g avertin + 15.5 mL tert-amyl alcohol). The mice in the control groups were intravenously injected with 100 µL of MEM-2 medium and the mice in the injected groups were intravenously injected with 100 µL of VHSV (2.37E+06 TCID50 · mL–1). Clinical signs and survival rates were observed for 14 days at room temperature. At the end of the experiment, the liver and spleen of the survivors were collected to determine the infection rate using a polymerase chain reaction (PCR) method (Snow et al., 2004).
Results and Discussion
Although VHS outbreaks have been reported at temperatures ranging from 2°C to 20°C, VHS usually occurs at temperatures between 4°C and 14°C (Isshiki et al., 2001; Kim et al., 2009; Kim et al., 2019). At temperatures between 15°C and 18°C, VHS usually takes a short course with modest accumulated mortality (Goodwin & Merry, 2011; Kim et al., 2019). Several previous studies showed that VHSV could survive outside the host for varying periods of time depending upon the physicochemical conditions of the aqueous medium, such as water temperature, organic materials, and salinity (Hawley & Garver, 2008; Kocan et al., 2001; Parry & Dixon, 1997). VHSV survived for longer periods at 4°C than at 20°C (Parry & Dixon, 1997), which agrees with the results of other studies (Hawley & Garver, 2008; Joiner et al., 2020). Nevertheless, Joiner et al. (2020) reported that VHSV suspended in MEM at 25°C could survive for 50 days, and Pham et al. (2011) showed that VHSV survived on the surface of the culture plate for 6 days at 37°C. VHSV could also replicate and cause CPE in mouse (BHK/21) and human diploid (WI-38) cells (Clark & Soriano, 1974). However, information on the survival of VHSV at a supra-optimal temperature (37°C) and the safety of the virus in mammals is limited.
In our study, VHSV titers (4.22E+06 TCID50 · mL–1) in MEM-2 without any cells at 15°C showed only one log reduction after 8 days, whereas the virus maintained at 37°C was inactivated after 8 dpi (Table 1). Similarly, Pham et al. (2011) found that VHSV bound to the surface of a 96-well cell culture plate at 37°C was inactivated after 6 dpi. Since the EPC cell line cannot survive and proliferate at 37°C (data not shown), the mammalian SNU-1411 and hepa-1c1c7s cells were used to determine the ability of VHSV to sustain and/or multiply at 15°C and 37°C. Although the optimal temperature for SNU-1411 and hepa-1c1c7s cells is 37°C, we found that the 15°C used in this study did not significantly affect their viability or condition. In general, mammalian cells can be stored at low temperatures (6°C–24°C) as an alternative short-term storage method (Hunt et al., 2005). In this study, VHSV was not detected in any negative control groups (data not shown). There was no recognizable CPE in VHSV-inoculated-SNU-1411 and hepa-1c1c7s cells incubated at 15°C and 37°C (Fig. 1). Although VHSV in mammalian cells was rapidly decreased at 37°C and eventually disappeared at 12 dpi, at 15°C, the virus was maintained up to 16 dpi (Table 2). Although whether or not VHSV can actually invade inside the cells is unclear, the TCID50 · mL–1 of VHSV in the 2 mammalian cells in this study was approximately 10 for up to 16 days, indicating that the virus could not multiply in the cells. As a previous study demonstrated that ovarian fluid significantly prolonged the survival and infectivity of VHSV (Kocan et al., 2001), the organic debris from mammalian cells might have influenced longer survival found in this study. The results obtained from our study are consistent with those of Samal et al. (1998), who reported that the SBR virus inoculated into mammalian-derived cell lines (Vero and bovine) at 35°C disappeared after 7 days, whereas it remained constant at 22°C. Washing the cells used in this study might not have been enough to remove the virus attached to the surface of the cells as other studies (Joiner et al., 2020; Pham et al., 2011; Yoshinaka et al., 2000) reported that washing and centrifuging procedures could not completely remove the virus attached to the surface of bacterial cells and other environmental matrices.
Temperature (°C) | Average viral concentration (TCID50 · mL–1) | |||
---|---|---|---|---|
Days post exposure (d) | ||||
0 | 1 | 4 | 8 | |
15 | 4.22E+06 | 3.51E+06 | 2.04E+06 | 3.51E+05 |
37 | 4.22E+06 | 8.55E+04 | 1.77E+03 | - |
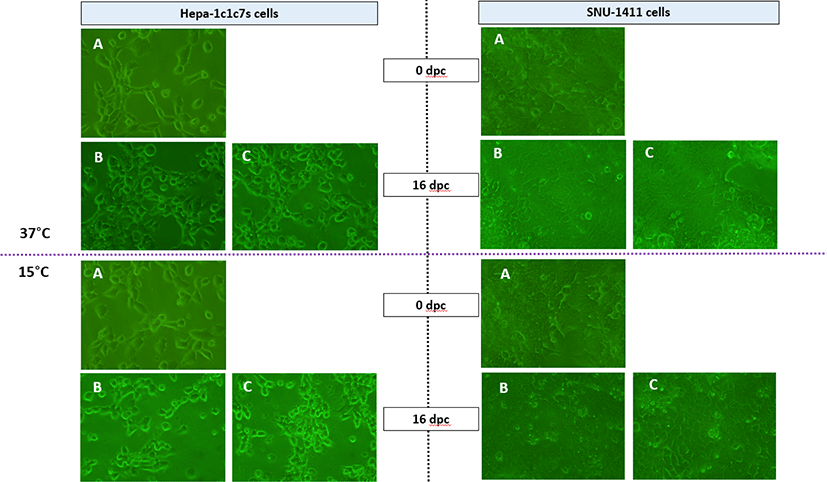
Although the tested mammalian cell lines did not permit VHSV replication at 37°C, the ability of VHSV to infect and maintain infectivity in a mammalian model has not yet been investigated. The in vivo experiment in this study found that mice at 14 days post-challenge did not show any clinical signs of disease or mortality, or any evidence of VHSV infection in the liver and spleen of the surviving individuals (confirmed by PCR, data not shown), indicating that it is highly likely that the virus injected into the mice was removed.
The result obtained from this study clearly showed that VHSV could be inactivated within a short period of time (4–8 days) when it was maintained at 37°C. Although this study also showed that VHSV could survive in mouse- and human-derived cell lines at 15°C, VHSV did not cause CPEs and replicate in the cells, or infect the mice. Therefore, in conclusion, it is highly unlikely that VHSV, which can infect a broad range of aquatic animal hosts, can infect mammals including humans.