Introduction
The mariculture of Pyropia yezoensis (a red seaweed) is a super prominent industry in East Asian countries, particularly in South Korea. The Korea’s annual production of dried P. yezoensis (nori or laver, and also called “Gim” in Korea) was over 14.0 billion sheets in 2021, obtained from 45,000 ha of marine space (Ale & Meyer, 2013; KAFFTC, 2021; Park & Hwang, 2014). Surprisingly, the exports of P. yezoensis to 120 countries in the same year surpassed 692.8 million dollars, which is an all-time high in Korea (MOF, 2022). However, the last year’s total output of P. yezoensis was decreased by 5.7% compared to the average output of the past five years (KAFFTC, 2021). The cultivation of P. yezoensis is hugely impacted by the diseases that appear under high temperature, especially red rot disease caused by Pythium porphyrae (Hwang & Park, 2020). The damage caused by the red rot disease is reported to be steadily increasing every year (Park & Hwang, 2014).
Gold 1 (G1) is a newly developed P. yezoensis in South Korea and registered in National Aquatic Plant Variety Center (Mokpo, Korea) as a strain highly resistant to red rot disease (AQ-22. June 29, 2021) (Kim et al., 2014; NIFS, 2021). G1 strain has higher production rate up to 30% and contains more protein than the commercially available strain of Pyropia yezoensis (CP) (MOF, 2020).
The moderate levels of free radicals perform the essential part of defense mechanism in organism, assisting the maintenance of innate immune system such as phagocytic destruction against bacterial infection. However, when excessive free radicals such as reactive oxygen species (ROS) are generated, they can adversely affect the host tissues and thereby contribute to the various pathological conditions such as cancer and skin damage with irreversible aging via disrupting of systemic antioxidant mechanism (Sharifi-Rad et al., 2020).
P. yezoensis is a popular seafood and has been traditionally used as a marine vegetable in Korea. It is a supreme nutritious substances containing 41% of proteins, 44% of polysaccharides, and 10% of fat and minerals (Bito et al., 2017) and has several beneficial effects such as anti-hypertension, anti-tumor, and anti-bacterial activities (Lee et al., 2015). According to the several studies, P. yezoensis and its active components has been proposed to be used as an excellent exogeneous antioxidant (Dai et al., 2020; Kim et al., 2018). To control systemic homeostasis, the development of antioxidant agent from the marine natural products would be promising approach for long-term use with safety (Heo et al., 2005). In the present, we performed a comparative study of antioxidant activities between G1 and CP to explore the functionality of a newly developed P. yezoensis.
Materials and Methods
G1 is kindly provided by Prof. Gwang-Hoon Kim (Kong-Ju National University, Gong-Ju, Korea). CP, which was matched spatiotemporal with the G1, was purchased from Seonjin Fisheries (Seocheon, Korea). Human embryonic kidney 293T (293T) cell line was purchased from the Korea Cell Line Bank (KCLB, Seoul, Korea). Dulbecco’s modified Eagle’s medium (DMEM), fetal bovine serum (FBS), penicillin-streptomycin, and trypsin-ethylenediaminetetraacetic acid were obtained from Gibco/BRL (Burlington, ON, Canada). All other chemicals and reagents were purchased from Sigma-Aldrich (St. Louis, MO, USA).
Preparation of CP and G1 water extracts was carried out by the procedure described previously, with a slight modification (Dai et al., 2020). Three gram of CP or G1 was soaked in 100 mL of de-ionized water, and the samples were shaken for 24 h at 35°C (120 rpm). The extracts were centrifuged at 1,900 ×g for 20 min, then the resulting supernatants were collected and filtered. Finally, the aqueous extracts were freeze-dried and stored at 4°C for the further experiment.
The proximate compositions (carbohydrates, protein, and polyphenols) of CP and G1 were analyzed by the official methods of analysis of the Association of Official Analytical Chemists (AOAC). The freeze-dried samples were resuspended in phosphate-buffered saline (PBS) (10 mg/mL), and their polysaccharides, protein, and polyphenol contents were determined by the colorimetric standard methods (Box, 1983; DuBois et al., 1956; Lowry et al., 1951). Glucose, bovine serum albumin (BSA), and gallic acid were used as a respective standard for the assays. All reactants were transferred to a 96-well plate and their optical density was measured at 480 nm, 540 nm, or 700 nm by using Synergy HT Multi-Detection Microplate Reader (BioTek Instruments, Winooski, VT, USA).
The antioxidant activity of the extract was analyzed by the measurement of 2,2’-azino-bis(3-ethylbenzothiazoline-6-sulfonic acid) (ABTS) or 2,2-diphenyl-1-picrylhydrazyl (DPPH) radical scavenging activities as reported previously (Heo et al., 2006). Briefly, for ABTS radical scavenging activity, 50 µL of the extract was mixed with equal volume of 0.1 M phosphate buffer (pH 5.0) in a 96-well plate, and 1/10 volume of H2O2 was added to the mixture. The samples were placed at 37°C for 5 min, then 15 µL of 1.25 mM ABTS and 15 µL of peroxidase (1 unit/mL) were applied to the mixtures. After incubation at 37°C for 10 min, the absorbance of the samples was measured at 405 nm. DPPH radical scavenging assay was carried out by the following method. Equal volumes of DPPH in methanol (4 × 10–4 M) and sample extract were combined and incubated for 30 min at room temperature. Then the absorbance of mixture was measured at 517 nm by using Synergy HT Multi-Detection Microplate Reader (BioTek Instruments).
293T cells were cultured in DMEM containing 10% FBS with penicillin/streptomycin (100 μg/mL) at 37°C under 5% CO2. To measure the ROS production and cell viability, 293T cells were seeded in 96-well plates at 1 × 105 cells/mL and cultured for 24 h. Then the cells were treated with various concentrations of CP or G1 diluted in PBS (250, 500, and 1,000 µg/mL) in the presence of 600 µL of H2O2 for 24 h. To measure the cell viability, 3-(4,5-dimethylthiazol-2-yl)-2,5-diphenyltetrazolium bromide (MTT) assay was used as described in the previous study (Cho et al., 2019). Intracellular ROS production was analyzed by using 2′,7′-dichlorofluorescin diacetate (DCF-DA) (Cho et al., 2019). To examine the antioxidant activities of CP and G1, the cells were treated with the extracts for 3 h and then with 300 µM of H2O2 for 1 h, thereafter the fluorescence intensity of the samples were determined at 485/520 nm (Excitation/Emission) for 24 h after the application of 5 µM of DCF-DA. The rate of apoptosis induced by H2O2 treatment was measured by Hoechst 33342 staining (Cho et al., 2019). In brief, the cells were treated with CP or G1 extracts for 1 h and then with 300 µM of H2O2 for 12 h. The H2O2-exposed cells were stained with 0.3 µM of Hoechst 33342 in PBS for 5 min in the dark. All the images of the stained cells were analyzed by fluorescence microscopy using Lionheart™ FX Automated Microscope (BioTek Instruments).
To analyze the changes in the expression of the apoptosis-regulating genes (Table 1), real-time quantitative reverse transcription polymerase chain reaction (qRT-PCR) analysis was performed as described in the previous study (Hyun et al., 2022). Total RNA of the cells was extracted by using TRIzol (Takara, Shiga, Japan) and used to synthesize cDNA by Moloney murine leukemia virus reverse transcriptase (Thermo Fisher, Waltham, MA, USA). qRT-PCR was conducted using a CFX 384 TouchTM Real-Time PCR Detection System (Bio-Rad, Irvine, CA, USA) with primers specific for target genes. The value of threshold cycle was confirmed using CFX ManagerTM (Bio-Rad). Beta actin (ACTB) was used as an internal control. The primer sequences used for the analysis are shown in Table 1.
Results and Discussion
Total production of Korean laver P. yezoensis, which accounts for about 70% of the world’s demand, has decreased over the past five years because of the prevalence of red rot disease (Hwang & Park, 2020). To solve this issue, a new strain of P. yezoensis, G1, with a strong resistance to red rot disease, has recently been developed and commercialized. Since CP is known to be a valuable source for various nutrients such as polysaccharides, protein and polyphenol (Dai et al., 2020; Kim et al., 2018; Meng & Geng, 2017), we compared the nutritional value of G1 with that of CP. The proximate compositions of the aqueous extracts are shown in Table 2. The extraction yield of G1 was significantly lower than that of CP (p < 0.05), though the difference range was minimal (38.67% vs. 34.67%). The contents of two major nutritional components, polysaccharides and protein, were much higher in G1 than CP (p < 0.05). Since it was shown that the contents of these water-soluble nutrients in P. yezoensis affect the flavor and taste (Hwang & Thi, 2014; Wang et al., 2022), it is likely that G1 has better sensory characteristics than CP. It was also reported that these macromolecules obtained by water extraction from P. yezoensis had a dramatic free radical-scavenging potency (Wang et al., 2022). On the other hand, the amounts of polyphenol in both extracts were much lower than that of other constituents, which is consistent with the previous reports (Dai et al., 2020; Nguyen et al., 2018), and there was no marked difference in the amounts between the samples (Table 2). Taken together, compared to the commercial one, a red rot disease-resistant G1 may not only have improved taste and flavor, but it is also expected to have higher nutritional quality.
Sample | Yield (%) | Proximate compositions (%) | ||
---|---|---|---|---|
Polysaccharides | Proteins | Polyphenols | ||
CP | 38.67 ± 1.33 | 46.08 ± 0.49 | 43.68 ± 0.22 | 1.45 ± 0.00 |
G1 | 34.67 ± 1.53* | 50.69 ± 1.03* | 51.41 ± 0.55* | 1.00 ± 0.23 |
Given the improvement of nutrient contents in G1, higher antioxidant activity of G1 can be expected (Sanjeewa et al., 2018). To compare the antioxidant capacity, the ROS scavenging activity of the extracts against DPPH+ and ABTS+ radicals were evaluated. As shown in Table 3, the half maximal inhibitory concentration (IC50) of G1 against DPPH+ free radical was relatively higher than that of CP, indicating less scavenging activity of G1 (803.84 ± 38.66 vs. 915.23 ± 20.81 µg/mL, p < 0.05). However, the scavenging activity against H2O2-induced ABTS+ radical was much more potent in G1 extract than CP (1,343.48 ± 99.93 vs. 588.10 ± 9.32, p < 0.05). It was shown that the free radical scavenging activity of water-soluble components in natural products can be measured more accurately by the H2O2-induced ABTS+ scavenging test compared to DPPH+ radical test (Floegel et al., 2011). Thus, the observation of improved antioxidant efficacy of G1 in ABTS+ free radical-scavenging assay suggests that G1 is relatively superior in radical scavenging ability compared to CP. Collectively, these results strongly indicate that, in addition to its nutritional predominance than CP, G1 has an improved antioxidant activity.
Sample | Free radical scavenging activity, IC50 (μg/mL) | |
---|---|---|
DPPH+ | ABTS+ | |
CP | 803.84 ± 38.66 | 1,343.48 ± 99.93 |
G1 | 915.23 ± 20.81* | 588.10 ± 9.32* |
Antioxidant activities of the extracts were further verified with H2O2-stimulated 293T cells, which are derived from human embryonic kidney tissue and widely used for the multiple biological studies associated with oxidative stress (Gyurászová et al., 2020; Jung et al., 2022; Li et al., 2020). First, the cytotoxicity of the extracts was examined in 293T cells in the presence of various concentrations of CP and G1 aqueous extracts (250, 500, and 1,000 µg/mL) using MTT assay. As shown in Fig. 1A, no cytotoxicity was observed for both extracts in the range of tested concentrations in 293T cells. To examine the cytoprotective effect of the extracts, the cells were treated with H2O2 in the presence or absence of the aqueous extracts. H2O2 treatment markedly reduced the cell viability to 43.38%, whereas this decrease was dramatically recovered by quercetin (QC) treatment up to 83.59%, which was used as a positive control for antioxidant activity (Fig. 1B) (Zhang et al., 2011). Interestingly, G1 extract also blocked the H2O2 -induced cell death at all concentrations of the treatment in a dose-dependent manner (Fig. 1B). The recovery rate at the highest concentration of G1 was almost identical to the extent of QC. CP extract showed the cytoprotective effect as well, while the range of effect was moderate and relatively lower than that of G1 (Fig. 1B).
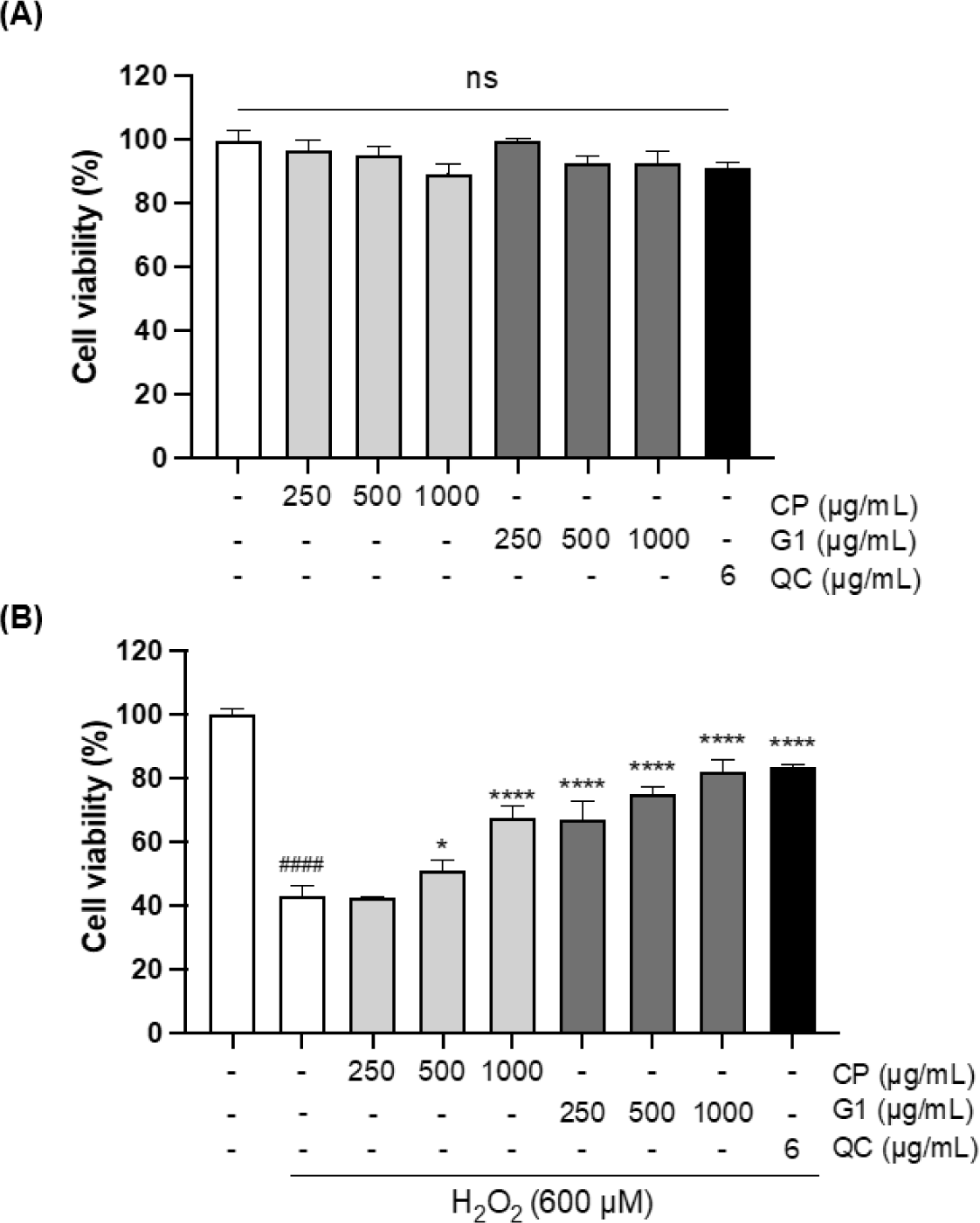
Exogeneous H2O2 treatment can not only boost ROS generation in mitochondria but also inhibit antioxidant enzymes such as manganese superoxide dismutase, glutathione peroxidase, catalase, and aconitase, leading to the oxidative stress in the cellular system (Arany et al., 2010; Miguel et al., 2009). To further explore the cytoprotective effect against H2O2 treatment, we evaluated the effect of the extracts on ROS production in H2O2-treated 293T cells. There was a marked increase in the formation of ROS by H2O2 treatment (p < 0.0001, Fig. 2). This increase was remarkably decreased by G1 pretreatment at all concentrations (p < 0.0001, Fig. 2), whereas the effect of CP was not observed (Fig. 2). Further quantitative comparison of IC50 also showed a significant difference in antioxidant activity between CP and G1 treatments (Fig. 2: inserted table, p < 0.05). These results suggest that the preventive effect on H2O2-induced cell death by G1 is associated with the decrease in intracellular ROS production, which alleviates the impact of exogenous H2O2 treatment.
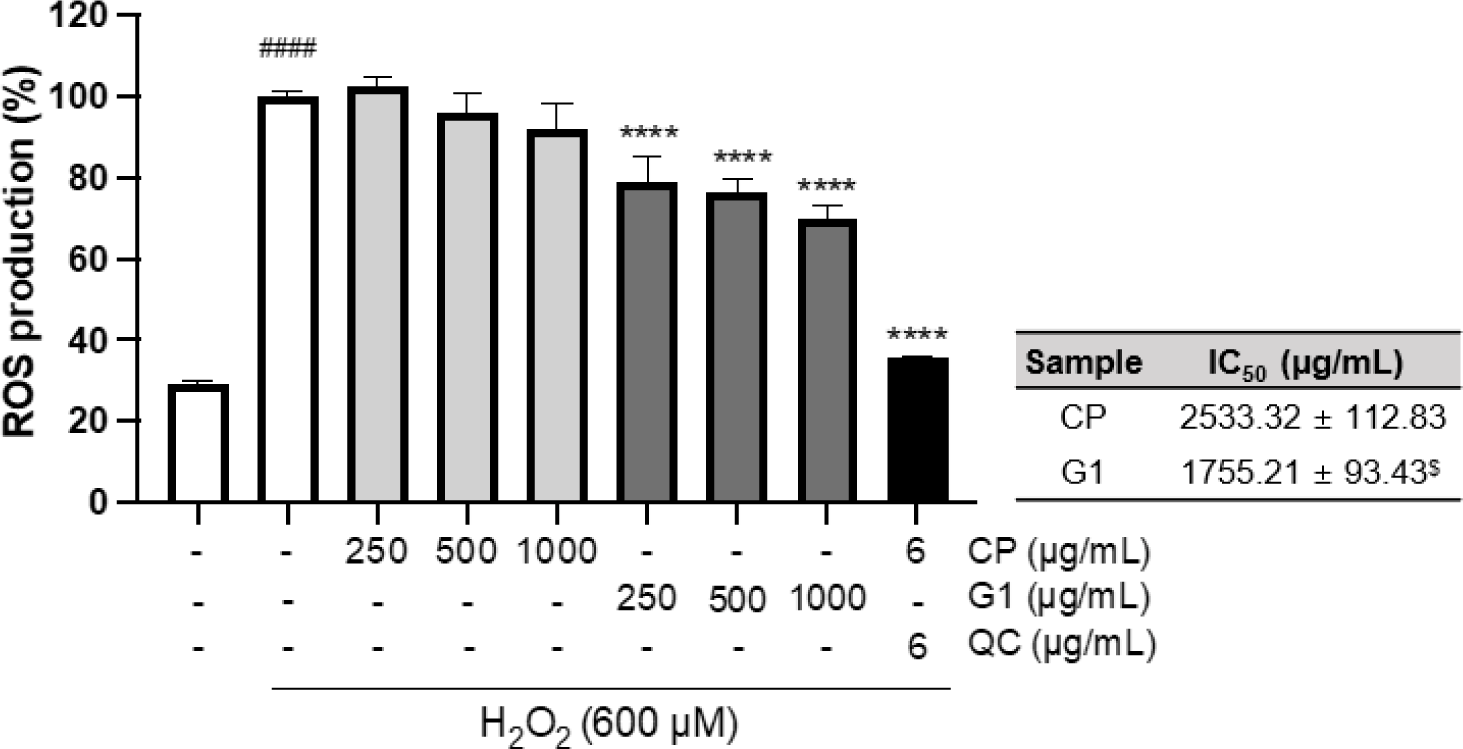
Previous studies have shown that the imbalance of redox system caused by either external ROS exposure or excessive intracellular ROS production can elevate the oxidative stress in the cell, which can lead to the apoptosis (Teramoto et al., 1999). We examined the apoptotic changes induced by H2O2 treatment in 293T cells and the effect of extracts pretreatment on those changes by fluorescence microscopy with Hoechst 33342 staining, which can classify healthy cells or apoptotic cells (Crowley et al., 2016). Treatment of the cells with H2O2 distinctly escalated the damages, including the chromatin condensation, DNA fragmentation, and the formation of apoptotic body (Fig. 3A). These changes were markedly diminished by both CP and G1 treatment in a dose-dependent manner (Fig. 3A). The quantitative analysis of these changes clearly indicates a critical mitigation of the apoptotic rate in both treatment groups (Fig. 3B). The protective effect was more potent in G1 compared to CP, as evidence by the comparison of IC50 (Fig. 3B: inserted table, p < 0.05). These results suggest that G1 extract effectively blocked H2O2 exposure-caused apoptosis via reducing endogenous ROS production in 293T cells.
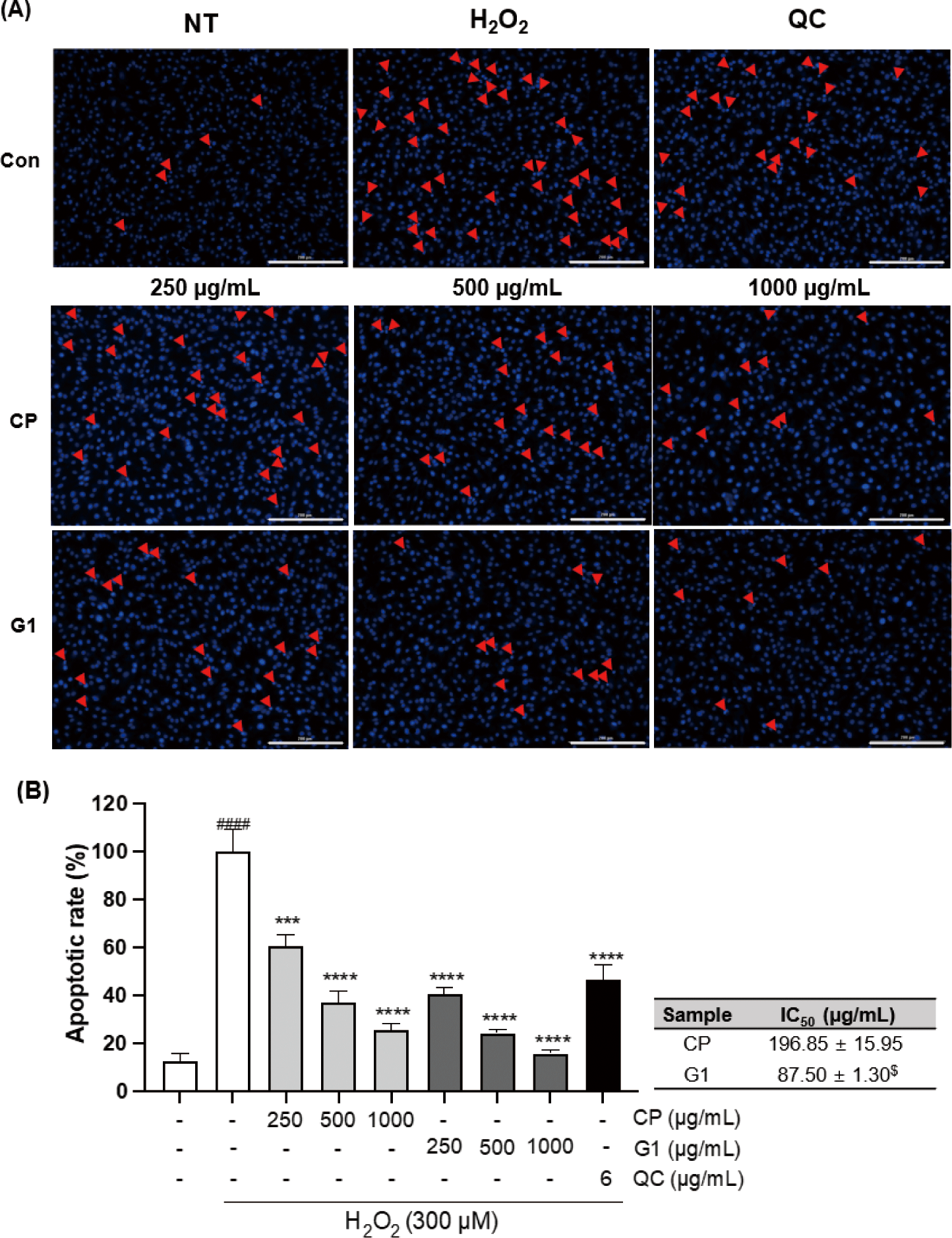
The DNA fragmentation due to the excessive oxidative stress can stimulate the expression of apoptosis-promoting genes (Zhang & Xu, 2000). To further verify the protective effect of the extracts on apoptotic changes in 293T cells, we examined the changes in the expression of various genes that are related with the regulation of apoptosis (Fig. 4).
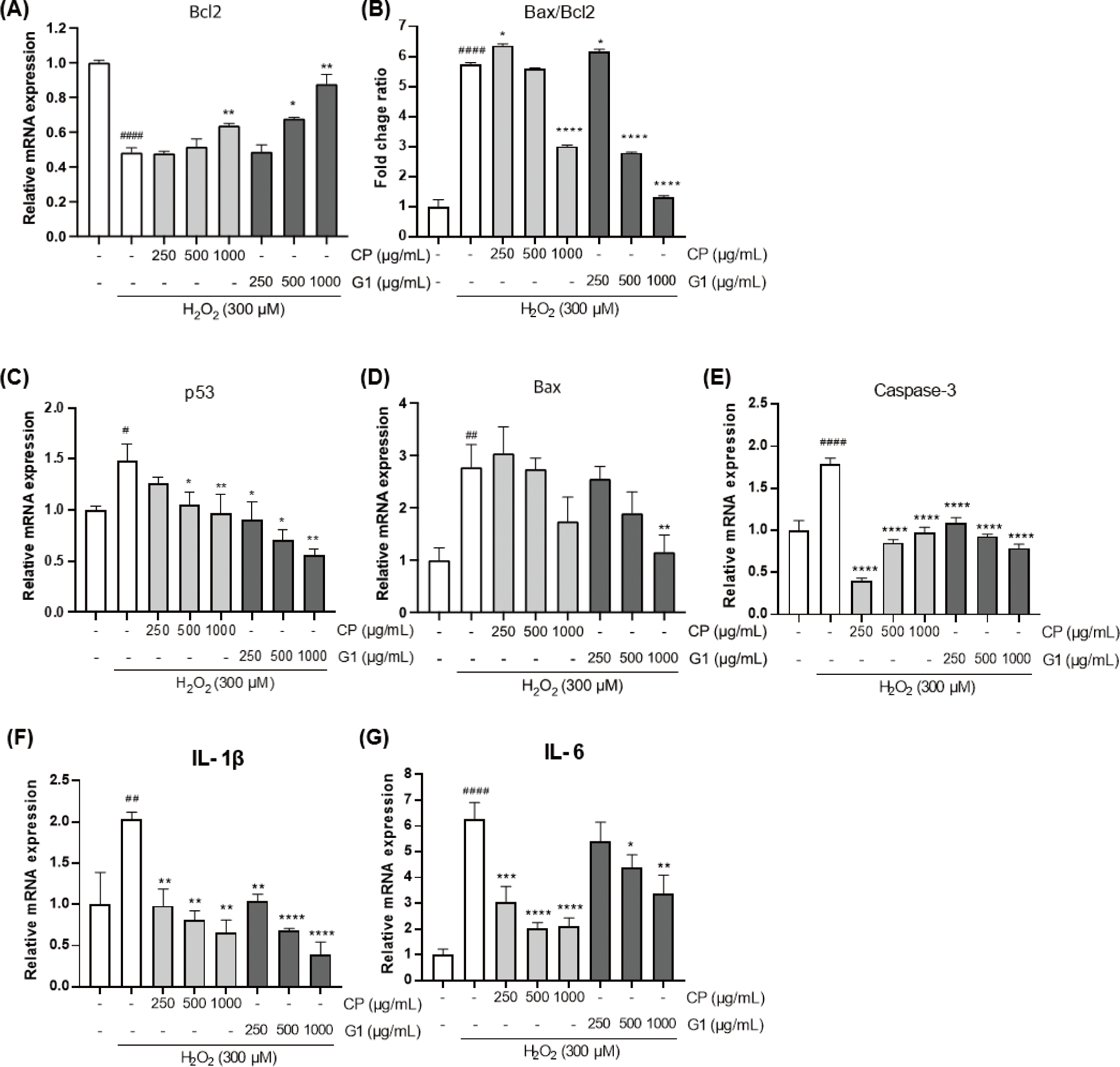
The expression of B-cell lymphoma 2 (Bcl2) gene was significantly declined by H2O2 treatment (Fig. 4A). This result is consistent with the previous report that showed the induction of apoptosis by H2O2 in 293T cells (Li et al., 2020) and indicates that H2O2-induced apoptotic cell model has been accurately implemented in the present study. Intriguingly, the H2O2-prompted decrease in Bcl2 expression was diminished by CP and G1 application. While CP showed a moderate effect on Bcl2 expression at the highest concentration, the G1 treatment showed more potent effect on the recovery of Bcl2 as evidenced by a dose-dependent increase with higher concentrations (Fig. 4A). Thus, this adjustment by G1 extract might control a cellular homeostasis through the cutting of pro-apoptotic signaling convey by the increase of Bcl2.
The expression of pro-apoptotic genes such as Bcl2-associated X protein (Bax), p53, and caspase-3, were remarkably increased by H2O2 stimulation compared to the non-treated cells (Fig. 4C–4E). Pretreatment of the cells with the extracts considerably downregulated the expression of those genes, and the inhibitory effect of G1 extract was more significant than CP (Fig. 4C and 4D). Tumor inhibitory p53 plays a pivotal role in regulating cell cycle at G1 stage and in inducing apoptosis, thereby quickly blunting the mitosis of tumor cells (Pitolli et al., 2019). Compared to CP, G1 showed more marked suppressive effect on p53 expression, particularly at higher concentrations (Fig. 4C). This result is consistent with the G1 extract-mediated increase in Bcl2 expression, which is one of the p53 target genes (Hemann & Lowe, 2006). As a Bcl2 family, Bax induces caspase-3 activation through cytochrome C release from mitochondria and is a key factor in the induction of apoptosis (Pitolli et al., 2019). Bax level was substantially declined in G1-treated group (Fig. 4D). In addition, caspase-3, an important executor of apoptosis, was significantly reduced in all doses of G1 compared to the H2O2-stimulated cells (Fig. 4E). It can be expected that the decrease in caspase-3 expression is closely associated with the marked reduction in both Bax expression and Bax/Bcl2 ratio in G1 cells (Fig. 4B, 4D and 4E).
Many studies showed that exposure of the cells to hydrogen peroxide is strongly involved in the activation nuclear factor-κB (NF-κB) along with its associated intracellular pathways, and the effective antioxidant can reverse this phenomenon (Oliveira-Marques et al., 2009; Sun et al., 2019). In the present study, the sole H2O2 treatment led to a dramatic increase in the expression of proinflammatory mediators such as interleukin (IL)-1β and IL-6 (Fig. 4F and 4G), which are well-known target genes of NF-κB (Hiscott et al., 1993; Son et al., 2008). These results may suggest that the expressions of those genes are increased via the activation of NF-κB pathway by H2O2 treatment. It was shown that IL-1β overexpression augments Bax/Bcl2 ratio and increases cytochrome C release from mitochondria, finally leading to apoptosis (Shen et al., 2017). The expression of IL-1β and IL-6 were considerably diminished in both G1 and CP-treated groups (Fig. 4F and 4G). Based on this finding, we confirmed that the inhibitory activity of G1 at the initiation of H2O2-induced apoptosis was also accompanied by the changes in the level of apoptosis-associated gene expression. In addition, a certain level of improvement in cell death was observed in CP-treated group as well.
Conclusion
In this study, a red rot disease-resistant strain of P. yezoensis, G1, showed a high nutritional value with outstanding antioxidant activity against hydrogen peroxide treatment as compared to CP. The in vitro assessment using 293T cells indicated that G1 has remarkable potential of protection against hydrogen peroxide-mediated cell death within the indicated concentrations range without any cytotoxicity. In addition, G1 specifically alleviated the endogenous ROS production induced by the exogenous hydrogen peroxide treatment of 293T cells. Furthermore, the elevation in the apoptotic rate induced by hydrogen peroxide stimulation was also substantially relieved by G1 treatment in a dose-dependent manner as compared to CP. With the decrease in the ROS production by G1 treatment, the expression of the genes related to the mitochondrial apoptotic pathway was dramatically recovered, and the integral apoptotic regulators were declined as well. Taken together, G1 may be used as a functional ingredient that reverses the free radical-induced cellular damage as an antioxidant source.