Introduction
Mesopelagic micronekton species are a community of organisms, typically ranging from 2 to 20 cm (Annasawmy et al., 2018) and swimming independently of ocean currents (Castellón & Olivar, 2023). Many of the pelagic organisms perform diel vertical migrations, from the mixed layers during night to deep layers during day (Benoit-Bird & Au, 2006). They play a major role in the pelagic ecosystem as food of large surface predators like tuna (Receveur et al., 2020). Micronektonic fish are regarded as key components of the micronekton (Klevjer et al., 2020). They are considered as the most abundant fish on Earth and most abundant vertebrates in the biosphere (Cherel et al., 2020).
The species Vinciguerria nimbaria, Jordan and Williams 1895 (Stomiiformes: Phosichthyidae) is a mesopelagic fish of the micronekton and widely distributed in Pacific (Clarke, 1978; Kawamura & Hamaoka, 1981), Atlantic (Shevchenko, 1986) and Indian (Gorbunova, 1982) oceans. In the tropic and subtropical oceans, V. nimbaria is found abundant at depths of 200 to 400 meters (Kim et al., 2023). In an equatorial area (10–20 °W, 0–5° N) of the Atlantic Ocean, a large purse-seine tuna fishery has occurred in a region classified as oligotrophic (Ménard et al., 2000). In these area, V. nimbaria feeds mainly on copepods (Champalbert et al., 2008; N’Goran & Pagano, 1999; Roger & Marchal, 1994) and in turn, this micronekton fish species constitutes the main prey of tunas, with more than 70% of the total preys ingested by these surface predators (Lebourges-Dhaussy et al., 2000; Roger & Marchal, 1994). According to Marchal & Lebourges (1996), the oceanic lightfish V. nimbaria instead of diving to a great depth during daytime as usual, remained in the upper layers and tunas were found to feed on them. In the equatorial area of the Atlantic Ocean, V. nimbaria is described as a zooplanktivore species (N’Goran & Pagano, 1999). In the same area, Champalbert & Pagano (2002) found that zooplankton biomass was high in summer during instability wave period and low in winter due to thermal stratification. The daily dietary requirements of V. nimbaria from the equatorial zone of the Atlantic Ocean have not been studied. Our main objective is to evaluate the daily rations in winter corresponding to the tuna fishing season and in summer, outside the tuna fishing season. The length-weight relationship (LWR) and length frequency distribution (LFD) are useful tools for fish ecology, fish resource assessment and fisheries management (Czudaj et al., 2022; Froese, 2006). Due to lack of information on the biometric growth parameters in V. nimbaria, this study is also conducted to determine the LWR and LFD in this oceanic lightfish.
Materials and Methods
The study was done during the production induite en zone de convergence par les ondes longues océaniques (PICOLO) research programme conducted by the French National Research, Institut de Recherche pour le Développement (IRD). The fishing region termed “PICOLO zone” includes the main tuna fishing zone (Fig. 1). The PICOLO 1 (P1) and PICOLO 3 (P3) surveys carried out on board of the oceanological vessel R/V ANTEA, respectively in boreal winter (January 17–February 10, 1997) and boreal summer (July 21–August 10, 1997). Winter is characterized by a marked thermocline and a weak divergence south of 0°30N (Champalbert & Pagano, 2002). It is also the tuna fishing period. Summer is defined by active divergence in the area and the propagation of instability wave (Morlière et al., 1994).
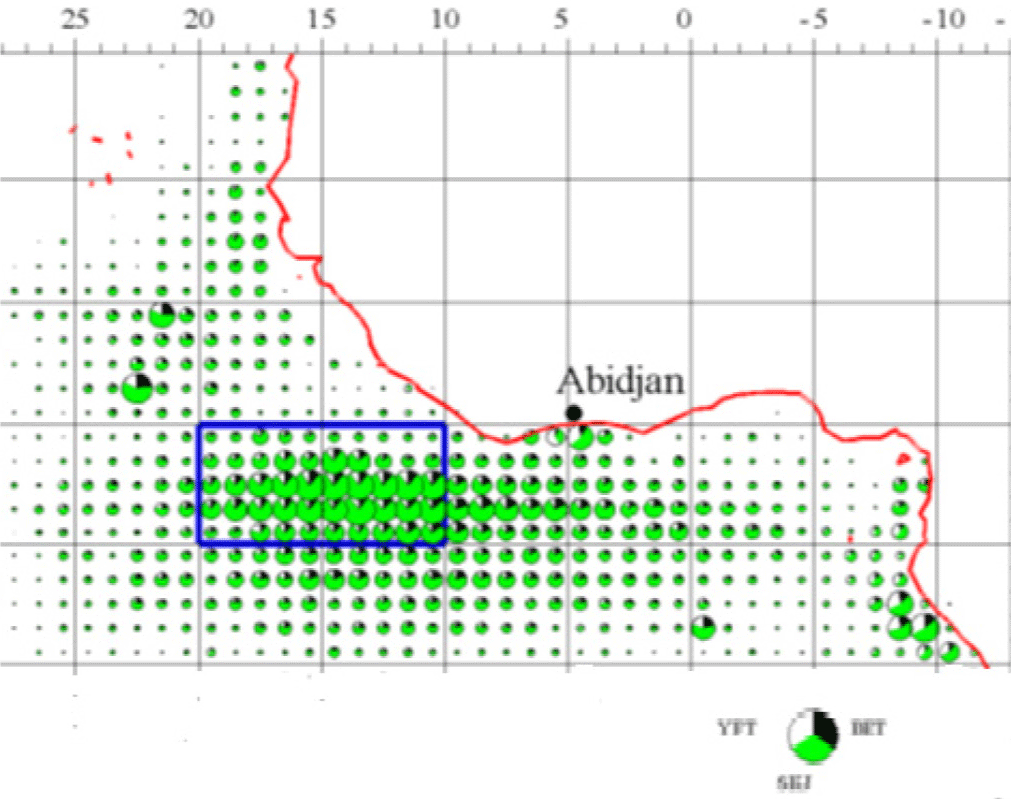
At each season, vertical profiles of temperature and salinity using a conductivity, temperature, depth probe (Seabird SBE 911) down to 160 m in winter and 250 in summer were recorded. Fish sampling is preceded by acoustic prospecting with the aim of detecting schools of Vinciguerria. nimbaria and determining fishing areas. Using a fixed Ossian dual-frequency (38 and 120 kHz) echo sounder, the reverberated volumes were calculated and the biomass of the organisms detected was estimated. A dual-beam dual-frequency sounder (Biosonics, Seattle, WA, USA) was used to determine the reflectance index of the targets and in particular that of V. nimbaria. Subsequently, the trawls were carried out in the high detection zones, with a pelagic trawl (opening 10 × 15 m; mesh of the distal part of the trawl: 10 mm2) coupled to a pressure sensor (Netsond, Furuno Electric, Nagasaki, Japan) making it possible to assess the fishing depth of the gear.
Data analysis
Two-way analysis of variance tests using OriginPro 2018 software (https://www.originlab.com/2018) were performed to test the effect of season and depth on temperature and salinity. Statistical analysis is performed for verification of significance (p < 0.05) on the results.
In each season, 7 trawls were carried out (winter [P1], at 2°N and summer [P3], at 0°). For each trawl, 20 fish taken at random were measured (standard length [SL]: snout end – caudal fin rays base) using a type 6902 mechanical caliper (accuracy: 0.05 mm) then weight with a Metler type scale (accuracy: 0.1 mg). A total of 280 fish specimens had their abdomens opened using a scalpel and a pair of scissors. The stomach after section at the pylorus was removed and weighed then emptied and weighed again to determine the weight of the stomach contents by difference between the two measurements. For the study of diet, each stomach content is preserved in a pillbox containing 5% borax-buffered formalin (Parsons et al., 1984). Later, the contents stomach of each fish is placed in a Dolfuss tank, followed by identification using determination keys (Grice, 1960; Trégouboff & Rose, 1957) and counting of the prey are carried out. Working on otoliths, Tomás & Panfili (2000), define larvae less than 15 mm SL, juveniles between 15–20 mm SL. In this study, size individuals between 20–30 mm were considered juveniles, those of size 30–38 mm were young adults and size > 38 mm adults.
Eggers (1977) model of calculating daily food intake is both simple and robust. Which makes it one of the most commonly used tools to estimate the daily needs of fish. The model of Eggers (1977) is an adaptation of the model of Bajkov (1935), which gives an estimate of the ration for 24 h (C24) from the relationship C24 = 24 × I24 × k. I24 is the mean repletion index in % and k the instantaneous rate of gastric evacuation is that of Worobec (1984), whose formula is as follows k = 0.0175 × T – 0.0442. This model assumes that the weight of food ingested is the same at the end and the beginning of the feeding cycle and the rate of gastric emptying is exponential. The fish samples collected are fresh until 9 PM. maximum, so we have a supply for 15 h maximum. Ration is calculated by the following formula C = 15 × I × k. The mean weight of the stomach contents and that of the fish makes it possible to calculate the repletion index which is involved in the calculation of the daily ration. Ir = WCs / WT, WCs mean weight of stomach contents and WT mean weight of fish. Ir is the repletion index and is expressed in % and characterizes the instantaneous state of filling of the stomach.
The LWR is analyzed by measuring the length and weight of collected fish samples using the cube formula (le Cren, 1951): W = aLb, where, W = weight of the fish (mg), L = standard length of V. nimbaria (mm), a = constant, b = exponential. The statistical significance of the “b” for the isometry (Ho: b = 3) was tested using Student’s t-test (α = 0.05). The isometric growth pattern (b = 3) means that the weight gain of the fish is balanced with the increase in length; b > 3 (positive allometric growth pattern) states that the weight gain of fish is faster than the length gain and b < 3 (negative allometric growth pattern) indicates that the weight gain of fish is slower than the length gain (Pramesthy et al., 2022). The power function is performed using Excel software for Windows version 15 (Microsoft, Redmond, WA, USA). The t-test made it possible to compare the length and weight parameters to the seasons and depth of this study. For the tests, we limited ourselves to adults of V. nimbaria due to their higher numbers in different seasons and depths.
Results
Fig. 2A and 2B showed the mean vertical profiles of temperature and salinity in winter (P1) and summer (P3). In winter, surface temperature was fairly homogenous (26°C) in the first 40 m. This layer is followed by a thermocline between 50 and 70 m, where the temperature suddenly varies rapidly. From 100 m, temperature (16°C) decreases more slowly. In summer, mean surface temperature was around 22°C. A weak decreasing temperature gradient appears from the surface to 100 m where the temperature around 15°C is followed by more stable layer. The two-way ANOVA (season and depth) shows a significant effect of season (p < 0.001) and depth (p < 0.001) on temperature, but the interactions between factors are not significant (p = 0.0608).
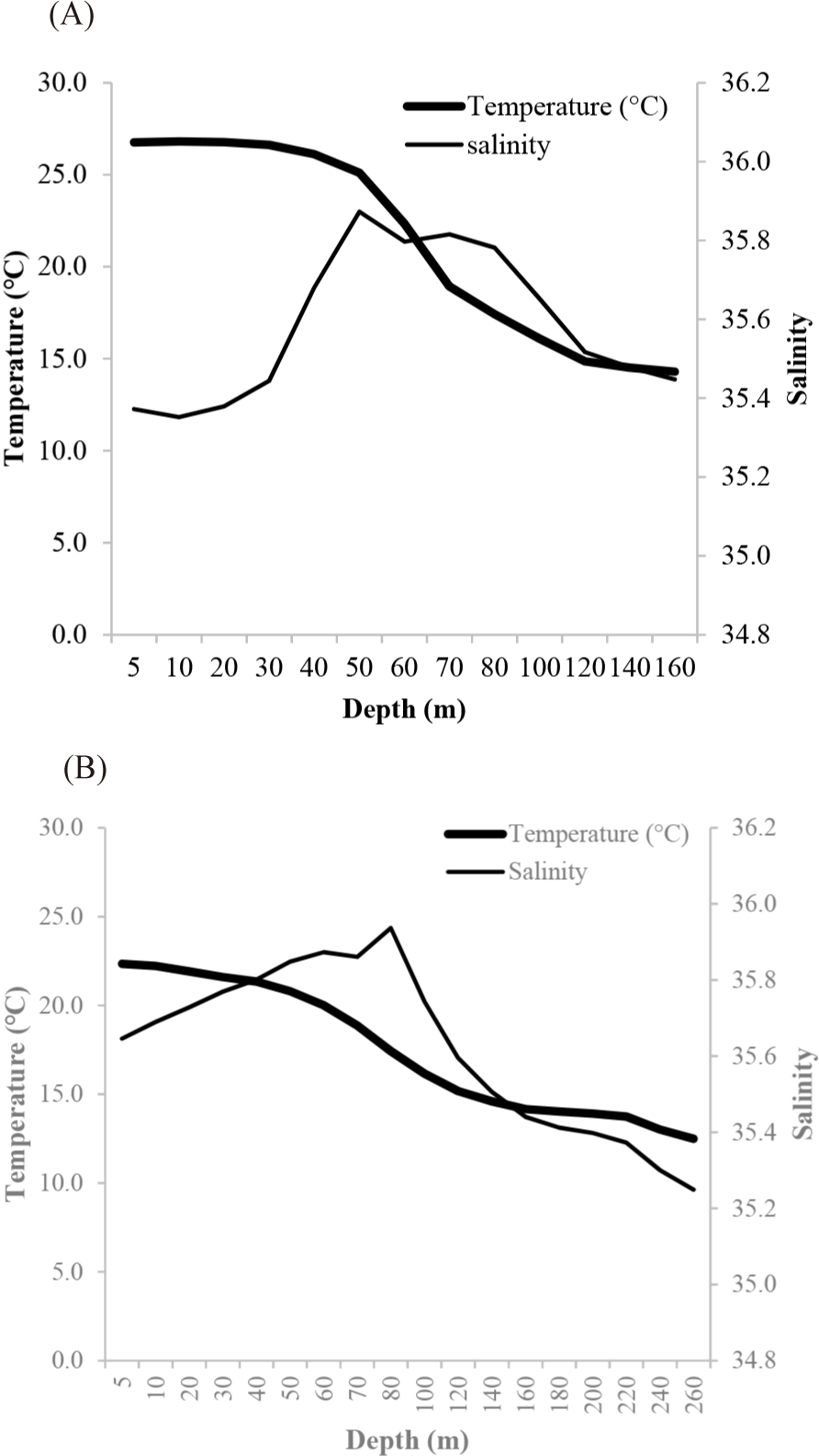
In both seasons, the salinity is between 35.2 and 36.0. The lower mean surface salinity in the surface layers increases with depth to reach its maximum between 50 and 70 m then gradually decreases. The two-way ANOVA (season and depth) shows no significant effect of season (p = 0.3255) or depth (p = 0.6590) on salinity.
Mainly, around ten families contain the species of zooplankton consumed by V. nimbaria in winter and summer. 2 to 5 families are numerically dominant with variations in percentage depending on age and fishing depth. In winter, the 140 stomach contents of this fish are dominated by 4 families (Oncaeidae, Corycaeidae, Clausocalanidae and Oithonidae) representing 86% to 95% of V. nimbaria prey. Two small copepods, notably Oncaeidae and Corycaeidae, constitute more than 70% of the diet whatever the age class. With 74% of non-empty stomachs representing 104 individuals, we have 10% young adults and 90% adults. The same prey on the surface (22–26 m) are found deeper, at the level of the thermocline (60–80 m; Table 1). In summer, the dissection of 140 fish from 7 trawls due to 20 fish per trawl highlighted the dominance of 8 families (Oncaeidae, Corycaeidae, Clausocalanidae, Calanidae, Euchaetidae, Scolecithricidae, Chaetognatha and Euphausiacea) depending on the age and depth of capture of fish (Table 2). Stomach contents reveal an emptiness rate of 34%. The 93 non-empty stomachs are represented by 15% juveniles, 35% young adults and 50% adults. Depending on the depth, we note that juveniles on the surface (31–40 m) feed more on small copepods while at depth (230–335 m) the prey are made up of a wide range of sizes. In young adults, the diet covers a wide size range with an increasingly large proportion of large prey which increases with depth. Adults seem to abandon small prey in favor of large prey both on the surface and at depth (Table 2).
In winter, daily ration estimates in surface waters are 2% higher than that at the thermocline. In summer, the ration of fish caught on the surface is twice as high as at depth. Both in summer and in winter, daily rations are higher on the surface than in the lower layers. At the same temperature (21°C), the daily ration is 2.2 times greater in summer than in winter (Table 3).
Seasons | Depth (m) | Temperature (°C) | Rate of gastric evacuation | Ration (% body weight) |
---|---|---|---|---|
Winter (P1) | 22–26 | 26 | 0.4108 | 9.2 |
60–80 | 21 | 0.3233 | 7.2 | |
Summer (P3) | 30–40 | 21 | 0.3233 | 16.0 |
230–335 | 12 | 0.1658 | 8.2 |
In winter (2°N), the fish numbering 140 individuals have a size between 35.9 and 50.0 mm, with a mean of 41.9 ± 2.6. They are made up mainly of adults (90%) and young adults (10%). In summer (0°), the size of the fish varies between 22.4 and 54.8 mm, for a mean of 37.8 ± 8.8. The distribution of the 140 fish gives 25% juveniles, 29% young adults and 46% adults. In winter, adults of V. nimbaria are smaller than those in summer and the mean weights vary in the same way (Table 4). The ‘t’ test gives p < 0.001. Indicating a significant difference in length and also weight between winter and summer. Taking into account the vertical distribution, on the surface as well as in the deeper layers, the sizes and weights of the fish are quite close (Table 5). Student’s t-test carried out on length and weight gives p = 0.606 and p = 0.105 respectively, reflecting non-significant differences depending on depth.
Season/parameters | SL (mm) | W (mg) | n |
---|---|---|---|
Winter | 42.1 ± 2.2 | 456.8 ± 59.1 | 128 |
Summer | 46.1 ± 4.8 | 855.8 ± 377.9 | 65 |
Depth/parameters | SL (mm) | W (mg) | n |
---|---|---|---|
Surface | 43.3 ± 3.2 | 549.8 ± 160.8 | 78 |
bottom | 43.5 ± 4.2 | 619.2 ± 353.3 | 115 |
The power function performed to study the LWR of fish collected during the two surveys is illustrated in Fig. 3. The relationship between the body weight (W in mg) and the SL (in mm) is computed as WP1 = 1.3477 × SL1.5563 (R2 = 0.5147) in the PICOLO 1 (P1) survey (Fig. 3A) and WP3= 0.0008 × SL3.6044 (R2 = 0.9256) for the PICOLO 3 (P3) survey (Fig. 3B). A negative (b < 3) allometric growth pattern is observed during the P1 survey, whilst a positive (b > 3) allometric growth pattern found for the P3 cruise.
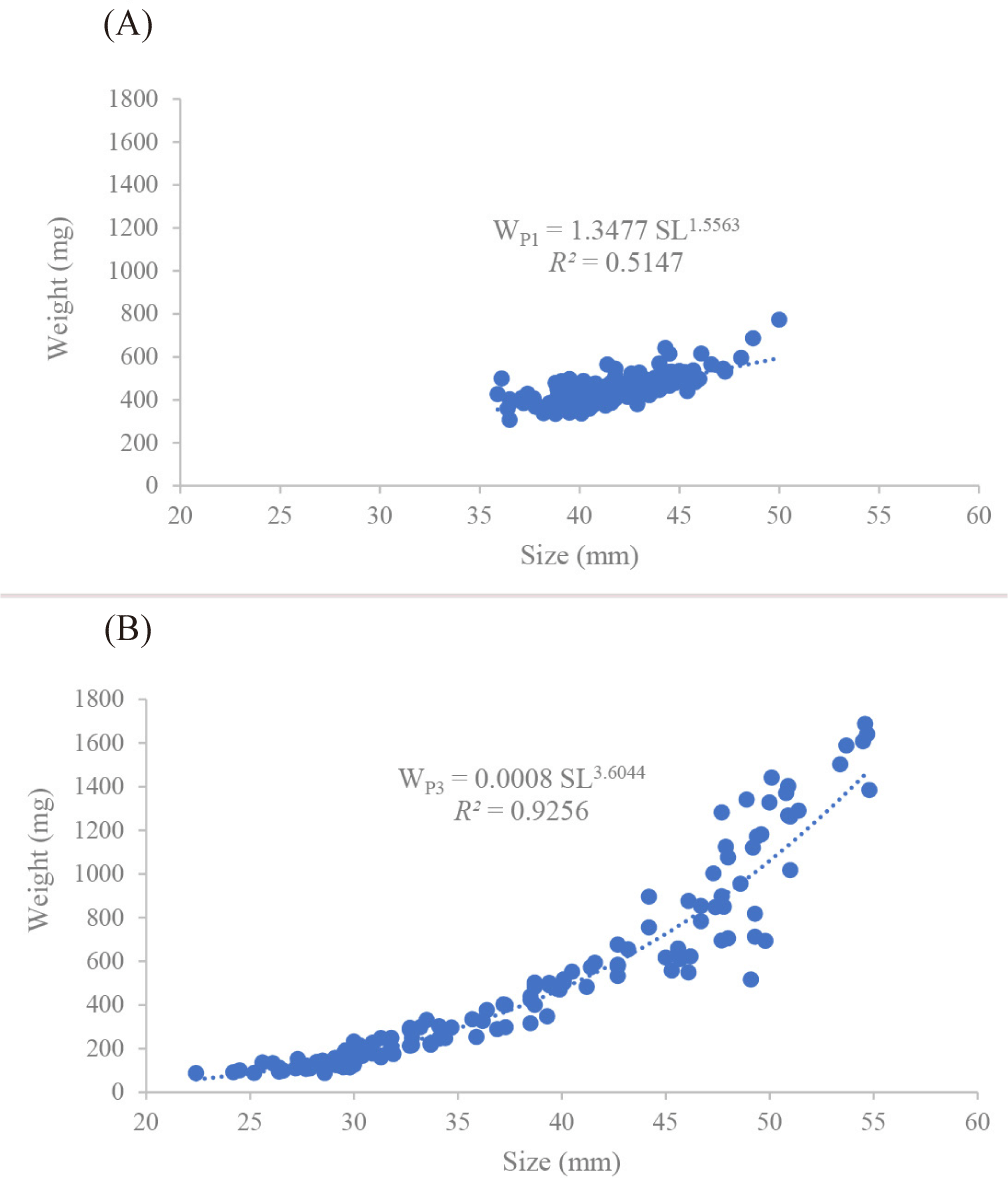
Discussion
The hydrology of the PICOLO zone in particular at latitude 2°N during the boreal winter was characterized by a marked thermal stratification with a homogeneous warm surface layer (> 26°C), followed by a thermocline below which the temperature decreases more slowly. On the other hand, in boreal summer, at the equator (0°) the area showed a weak thermal gradient with a mean surface temperature 4°C lower than that found in surface waters in winter. This drop in temperature results from a rise of deep, cold water (upwelling). Our observations are in agreement with those Champalbert & Pagano (2002) and Menkes et al. (2002), indicating the existence of an active divergence at the equator in summer.
The bulk of the food of V. nimbaria was dominated by copepods as this group represented 87%–95% of prey in winter and 16%–80% in summer. These variations in copepods between winter and summer could be linked to the very high percentages of indeterminate prey due to their state of advanced digestion during the summer. Many studies in Indian (Kalinina & Shevchenko, 1984; Legand & Rivaton, 1969), Pacific (Clarke, 1978; Kawamura & Hamaoka, 1981; Ozawa et al., 1977) and Atlantic Oceans (Champalbert et al., 2008; Lebourges-Dhaussy et al., 2000; N’Goran & Pagano, 1999; Shevchenko, 1986, 1996) attest that this small pelagic species is zooplanktivorous. Notable differences in diet were observed in the two seasons. In boral winter (P1), young adults and adults feed mainly on small prey such as Clausocalanidae, Oncaeidae and Corycaeidae. On the other hand, during the boreal summer (P3), the diet is more varied with a preponderance of large prey composed in particular of Copepods (Calanidae, Scolecithricidae, Euchaetidae), Chaetognatha and Euphausiacea. It has been noted that the size of the prey increases with the size of the fish. Thus, although feeding on large zooplankton organisms including copepods, juveniles consume more small-sized prey unlike adults who abandon small-sized prey for medium- and large prey. This seems to indicate in adults, a more efficient sequence of pursuit, attack and capture of large prey. Our results showed that in summer, adult fish are larger and heavier than in winter. Which seems to indicate a better state of well-being in summer than in winter. Furthermore, the more diversified and larger prey in summer than in winter reflects a more favorable environment in summer than in winter where the mean surface temperature 4°C lower than that of winter seems to be linked to cooling by the rise of cold waters at the equator. Indeed, the enrichment due to equatorial upwelling which manifests itself by a cooling of the surface layers favors the development of the trophic chain. So, food conditions are more favorable in summer (P3) than winter (P1) with a higher proportion of large prey. According to Shevchenko (1986), in regions of high productivity, prey from the stomachs of V. nimbaria show greater diversity than those found in areas of low productivity where the qualitative composition of the prey consumed is little varied.
Our results indicate that for the same temperature (21°C), daily rations are significantly greater in summer than in winter. This could be explained by the size, quality and variety of prey in summer unlike in winter where preys are small and their diversity is rather low. Our daily ration values in summer are close to that of Clarke (1978) in the Pacific in April-July giving a daily ration of 18% in juveniles. Kawamura & Hamaoka (1981) during the same period give in adults of V. nimbaria (38–54 mm) a relatively lower ration (10.5 ± 3.9%). The particularly significant predatory action of V. nimbaria was highlighted by Legand & Rivaton (1969) who estimated the weight of the stomach contents in relation to the weight of its body at 5%. This voracity appears more in relation to the rations of related species, notably Lampanyctus alatus (2.4%–4.4% of W) in the Gulf of Mexico (Hopkins & Baird, 1985). Dalpadado & Gjøsaeter (1988) reported rations of the order of 4.5% of W. This fish is therefore a voracious predator, which in winter feeding on small surface prey seems to require more time to cover daily food needs. This leads to favorable trophic conditions for the tuna, which congregate in this sector, thus creating a special fishing area.
In boreal winter V. nimbaria exhibited negative (b < 3) allometric growth pattern. This indicates that the weight gain of fish is slower than the length gain (Pramesthy et al., 2022). But, during an instability wave period, the growth pattern of the fish is positive allometric (b > 3). This means that at this period of the year (boreal summer), the increase in fish body length is slower than the fish W (Dewiyanti et al., 2020). When the allometry is positive, it reflects a state of well-being for the species in its environment which tends to gain weight. Which seems to indicate that she has everything she needs for her development. This trophic satisfaction results in being overweight. Which is probably the case in boreal summer. This difference of allometric growth pattern of V. nimbaria observed between boreal winter and boreal summer can be explained by the quality and weight of the biomass of the prey consumed. Large preys have a comparatively greater biomass than small preys. Insufficient energy requirements result from insufficient nutritional caloric intake from prey. We clearly observed that in summer there is a clear dominance of large prey unlike in winter where preys are mainly small. Razouls & Razouls (1978) attest that, from an energetic point of view, the nutritional quality of water bodies depends much more on the available zooplankton biomass than on the calorie content. They indicate that in the neritic zone of the Mediterranean, calorific values characterize “lean” zooplankton dominated by small species unlike typically oceanic zones offering a diet of richer energy value. Consequently, energy needs are met in summer, but in winter supplies remain insufficient.
Conclusion
During both seasons, mean surface temperature was 4°C higher in winter than in summer, with more marked thermal stratification. In winter, the food composition, mainly made up of small copepods, is the same in both young adults and adults. In summer, the size of the prey increases with the age of the fish. Juveniles consume more small prey than young adults and adults who concentrate on medium and large prey. More diverse prey reflects more favorable trophic conditions in summer than in winter.
The daily ration estimated for the two periods of the year gives values twice as high in summer as in winter at 21°C in surface marine waters. LWRs show in winter that fish grow faster than they gain weight, negative allometry (< 3). In summer, on the other hand, they gain weight faster than they grow, positive allometry (> 3). This positive allometry is indicative of a state of well-being in its environment. Since the study was carried out over two seasons, it would be interesting to cover the other two seasons to see what happens in autumn and spring regarding rations and trophic activities.