Background
Due to the paucity of oceanic resources utilized in the preparation of diets for cultured fish, commercial feed producers have been trying to replace fishmeal (FM) by using alternative protein sources such as vegetable protein meals (VMs) (Tacon and Metian 2008). Vegetable products include soy, canola (rape), corn, wheat, cottonseed, lupin, sunflower, flax linseed, and peas. Of these, soybeans are promising because of their higher protein content, higher digestibility, and better amino acid profile in comparison to other grains and oilseeds (Hardy 1999; Gatlin et al., 2007; Zhang et al., 2014).
The main drawbacks of using plant-derived proteins in fish feed are related to the low level of indispensable amino acids (in particular, methionine and lysine) and to the presence of a wide variety of anti-nutritional factors, such as saponins, lectins, phytate, trypsin inhibitors, phenols, and tannins (Francis et al. 2001), which could damage the intestinal tract thus reducing nutrient absorption and fish growth (Van den Ingh et al. 1991; Knudsen et al. 2008; Urán et al. 2008a, b).
Indeed, studies on Atlantic salmon (Salmo salar), rainbow trout (Oncorhychus mykiss), common carp (Cyprinus carpio), and gilthead sea bream (Sparus aurata) have indicated that the inclusion of less-refined plant products such as soybean meal (SBM) in the diet triggers an inflammation process in the distal intestine, referred to as SBM-induced enteropathy (Baeverfjord and Krogdahl 1996; Olli and Krogdahl 1994; Van den Ingh et al. 1991; Knudsen et al. 2008; Urán, et al. 2008a, b; Venou et al. 2006). Although the provoking mechanism remains to be established, the SBM-induced enteritis is believed to be caused by a disruption of the intestinal barrier, with subsequent exposure of otherwise shielded layers of the mucosa to luminal ingredients, including food-derived and microbial antigens (Romarheim et al. 2011). The typical signs of such inflammation are a shortening of the primary and secondary intestinal mucosal folds, an increase in the number of Goblet cells, and the infiltration of inflammatory cells, particularly macrophages and eosinophilic granulocytes into the lamina propria. This situation reduces the capacity of the enterocytes lining the epithelium to absorb nutrients (Baeverfjord and Krogdahl 1996; Van den Ingh et al. 1991; Buttle et al., 2001). These effects proved to be dose-dependent in Atlantic salmon; the worst symptoms were observed at the highest inclusion level (30%), but even diets containing as low as 7.6% SBM produced morphological changes at the intestinal level (Krogdahl et al. 2003).
It has been well documented that the responsible for the off-target effects of SBM is not the soy protein but other components present in the SBM, such as the anti-nutritional factor saponin, in combination with at least one or more unidentified components (Van den Ingh et al. 1991; Baeverfjord and Krogdahl 1996; Bakke-Mckellep et al. 2000; Krogdahl et al. 2003; Knudsen et al. 2008).
Once an inflammatory immune response is initiated, proinflammatory cytokines and a panel of chemokines (secreted proteins that play a major role in the inflammatory response) instigate the coordinated expression of downstream genes (Martin et al. 2006; Kortner et al. 2012; Grammes et al. 2013; Sahlmann et al. 2013; Marjara et al. 2012; Krogdahl et al. 2015; De Santis et al. 2015). The expression of pro- and anti-inflammatory cytokine genes was quantified by Urán et al. (2008a) in the isolated intraepithelial lymphocytes of common carp (Cyprinus carpio L.), in which an intestinal inflammation was observed. The enteropathy was developed when carp continuously fed on animal protein were transferred to a diet in which 20% of the protein was replaced by SBM. After 3 weeks of feeding, the pro-inflammatory interleukin 1β (IL-1β) and tumor necrosis factor α1 (TNF-α1) genes were upregulated whereas the anti-inflammatory interleukin 10 (IL-10) was downregulated after an initial upregulation at the first week of feeding.
The effects of replacement of FM with VM, often accompanied by reduced fish performance, are not restricted to SBM inclusion solely but have been observed after inclusion of many plant protein sources in several teleost species such as gilthead sea bream, turbot, Atlantic cod, and parrot fish (Gomez-Requeni et al. 2004; Sitja-Bobadilla et al. 2005; Yun et al. 2011; Hansen et al. 2007; Lim and Lee 2009). Baeza-Ariño et al. (2014) described liver and gut alterations of gilthead sea bream, S. aurata L., fed on diets in which FM was replaced by a mixture of rice and pea protein concentrates. The results of the histological analysis showed significant changes in the case of the 90% substitution in parameters such as thickness of the gut layers, number of Goblet cells, and villi’s length and thickness, whereas the integrity of the gut structure was not significantly affected by a diet with up to 60% of replacement. In some cases, severe vacuolization was encountered, which consequently deformed enterocytes and displaced the nucleus.
The short chain fatty acid of butyrate may promote the healing of inflamed intestine through its major role in enhancing epithelial cell proliferation and differentiation and in improving the intestinal absorptive function (Canani et al. 2012; Gálfi and Neogrády 2002; Wong et al. 2006). Like other short chain or volatile fatty acids (acetic, propionic, valeric, and caproic), butyric acid is produced during the fermentation of dietary fibers by the anaerobic microbiota associated with the epithelium of the animals’ digestive tract. In addition to being the main respiratory fuel source of the intestinal bacteria, and preferred to glucose or glutamine, this four-carbon chain organic acid molecule has potential immunomodulatory and anti-inflammatory properties (Vinolo et al. 2009; Toden et al. 2007; Terova et al. 2016), and exert multiple other beneficial effects on host energy metabolism (Hamer et al. 2007; Den Besten et al. 2013; Liu et al. 2014; da Silva et al. 2016). Although the mechanisms underlying these effects are still enigmatic and subject of intense scrutiny, it is believed that they encompass the complex interplay between diet, gut microbiota, and host energy metabolism.
However, much of the research on butyrate has been focused on terrestrial vertebrates, including humans, while very few studies have been conducted in fish. In particular, little is known about the effects of butyrate used as a feed additive on fish intestinal integrity. In terrestrial farmed animals such as pig and chicken, butyrate included in the diet has had a positive influence on body weight gain, feed utilization, and composition of intestinal microflora. It exerted trophic effects on the intestinal epithelium through an increase in the villi length and crypt depth, too (Gálfi and Bokori 1990; Kotunia et al. 2004; Hu and Guo 2007). In fish, Robles et al. (2013) reported an effect of butyrate used as a feed additive in increasing the availability of several essential amino acids and nucleotide derivatives, which have been demonstrated to increase fish growth when they are added individually to the diet.
Another feed additive that has been shown to have a restorative effect on soya saponin- and soya lectin-induced enteritis in fish (Iwashita et al. 2008, 2009) is the organic acid taurine. A number of recent studies reviewed by El-Sayed (2014) and Salze and Davis (2015) have demonstrated the essentiality of dietary taurine for many commercially relevant cultured species, including marine teleosts. According to these studies, taurine is involved in many physiological functions in fish and represents an essential nutrient, which exerts powerful antioxidant and anti-inflammatory properties, and is required as a supplement in the feed when a relevant percentage of vegetable protein sources are utilized. Taurine is a neutral β-amino acid found in high concentrations in animal tissues, whereas plants contain less than 1% of the taurine levels found in animals. In mammals, taurine is mainly synthesized in liver and brain through enzymatic oxidation and direct conversion of cysteine (derived from methionine) to cysteine sulfinic acid and hypotaurine. In this pathway, the enzymes cysteine dioxygenase (CDO) and cysteinsulfinate decarboxylase (CDS) have important roles (Griffith 1987; El-Sayed 2014). Therefore, in animal taxa with limited or no activity of the CDS, such as marine fish species, a continuous supply of taurine should be provided with the diet (El-Sayed 2014; Johnson et al. 2015; López et al. 2015). Indeed, Yokoyama et al. (2001), by comparing the CSD activity and hypotaurine production in several teleost and mammalian species, found that the only fish species with a high CSD activity were rainbow trout and tilapia. However, even in these species, the enzyme activity was an order of magnitude lower than that found in mammalian species. Other data (Salze and Davis 2015) indicate that both species benefit from dietary taurine supplementation thus suggesting that levels of CSD activity are insufficient to provide the necessary amounts of taurine for maximum growth of these species.
Accordingly, the objective of the present research was to evaluate the mitigating effects of butyrate and taurine used as feed additives on the morphological abnormalities caused by a SBM-based diet in the intestine of European sea bass (Dicentrarchus labrax). In order to attain this aim, we utilized both light and transmission electron microscopy (TEM) to determine intestinal changes in fish. We also quantified the intestinal messenger RNA (mRNA) abundance of several genes involved in the mucosal inflammatory response such as TNFα, which is a cell-signaling protein (cytokine) that makes up the inflammatory acute-phase reaction, and interleukins such as IL-8, IL-1β, IL-10, and IL-6, which are well-known cytokines that regulate immune responses, inflammatory reactions, and hematopoiesis.
Methods
The feeding experiment was conducted at the water recirculating system facility of the Department of Biotechnology and Life Sciences, University of Insubria, Varese, Italy. After being individually weighted and tagged, 30 European sea bass (D. labrax) of an average weight of 514 ± 67.4 g were transferred into six circular 750-L tanks with 5 fish/tank for 1-week acclimation before the start of the feeding trial. During the feeding trial, fish were fed two times a day at apparent satiety with three different diets (Table 1) used in duplicate (two tanks per diet).
SPC soy protein concentrate, DCP dicalcium phosphate
aMixed oil: 40% corn 60% soy
bVitamin and mineral premix (quantities in 1 kg of mix): vitamin A, 4,000,000 IU; vitamin D3, 800,000 IU; vitamin C, 25,000 mg; vitamin E, 15,000 mg; inositol, 15,000 mg; niacin, 12,000 mg; choline chloride, 6000 mg; calcium pantothenate, 3000 mg; vitamin B1, 2000 mg; vitamin B3, 2000 mg; vitamin B6, 1800 mg; biotin, 100 mg; manganese, 9000 mg; zinc, 8000 mg; iron, 7000 mg; copper, 1400 mg; cobalt, 160 mg; iodine 120 mg; anticaking and antioxidant + carrier, making up to 1000 g
Fish were sampled at the end of the feeding experiment, which lasted 60 days. The experimental tanks were connected to a 20-m3 water recirculation system. The water parameters such as pH, temperature, and dissolved oxygen (DO) were strictly controlled during all the experiment. Temperature was maintained at 20 ± 0.5 °C; salinity at 22 g L−1; pH 7.2; total N–NH3 ≤ 0.1 mg L−1; N–NO2− ≤ 0.02 mg L−1; N–NO3− ≤ 5 mg L−1, DO 8–8.5 mg L−1, and DO saturation, over 97%.
Fish were euthanized by an overdose (320 mg/L at 22 °C) of anesthetic (tricaine-methasulfonate MS-222, Sigma-Aldrich, Italy) and then weighed individually. The specific growth rate (SGR), the feed conversion ratio (FCR), and the condition factor (K) were calculated using the following formula:
The distal intestine of all sampled fish (six fish/diet, three from each tank) was dissected out. A small part of it was fixed in 4% buffered formalin, pH 7.2, for histological, immunohistochemical or electron microscopy examination, whereas the remaining part was conserved at –80 °C for gene expression analyses.
We used three experimental diets (C, B, and T), which contained the same low percentage of fishmeal and high percentage of soybean meal, as shown in Table 1. Diets B and T were supplemented with 0.2% of sodium butyrate or taurine, respectively, whereas Diet C, which represented the control diet, was not supplemented.
Sampled intestines were fixed by immersion in 4% buffered formal, dehydrated with a graded ethanol series (20, 30, 50, 70, and 95%), embedded in paraffin, and sectioned at 4 μm. After dewaxing and rehydrating the sections, they were consecutively stained with hematoxylin-eosin. Periodic Acidic Schiff (PAS) and Alcian blue stain at pH 2.5 were used to reveal neutral and acidic mucosubstances, respectively.
The thickness of intestinal wall was measured by analysing six intestines/diet and using the average value obtained from 3 tissue sections/intestine (n = 6). The length of muscle layer and mucosa was measured (in micrometers) by using the Image J software package and then the percentages were calculated by referring to the thickness of the whole intestine wall. Statistical differences were calculated by ANOVA followed by Duncan’s post hoc test, and P < 0.05 was considered as level of significance. Statistical analysis was performed using Statistica 7.0 software (StatSoft Inc., Tulsa, OK, USA).
Paraffin sections of distal intestine were dewaxed and rehydrated with a standard step of decreasing ethanol series. After washing with PBS, sections were pre-incubated for 30 min in blocking solution (2% bovine serum albumin, BSA, 0.1% Tween in PBS) and then incubated overnight at 4 °C with the polyclonal antibody rabbit anti-human CD45 (GenScript NJ, USA) diluted 1:100 in blocking solution. The washed specimens were incubated for 1 h at room temperature with the secondary antibody goat anti-rabbit (dilution 1:100 in PBS/BSA) conjugated with alkaline phosphatase (Jackson, Immuno Research Laboratories, West Grove, PA, USA). Secondary antibody alkaline phosphatase conjugated was visualized using 5-Bromo-4-Chloro-3-Indolyl Phosphate/Nitro Blue Tetrazolium tablets (BCIP/NBT, Sigma). Mounted slides were examined under an optical microscope (Nikon Eclipse Ni, Nikon, Tokyo, Japan). Images were acquired with a DS-5 M-L1 digital camera system (Nikon). Control sections were incubated in PBS/BSA without the primary antibody. To avoid false positives due to the chromogen reaction with endogenous alkaline phosphatase, sections were treated with CH3COOH 20% applied for 15 min at 4 °C.
Specimens already fixed in formalin were transferred to a 2% Karnovsky solution at 4 °C for 2 h, post-fixed in osmium tetroxide for 1 h, and then embedded in Epoxy resins. Ultra-thin sections were stained with uranyl acetate and lead citrate and examined with a TEM Morgagni Philips/FEI electron microscope.
Total RNA was extracted from 125 mg of sea bass distal intestine (6 fish/diet). Tissue lysis and homogenization were performed in special disposable sterile tubes (GentleMACS M tubes™, MiltenyiBiotec), in order to minimize the possibility of cross-contamination between samples, and using the gentleMACSDissociator (MiltenyiBiotec). After an automated purification process by using the Maxwell® 16 Instrument and Maxwell® 16 Tissue LEV total RNA purification Kit (Promega, Milan, Italy), the RNA was isolated. This RNA purification procedure provides an easy method for efficient, automated purification of highly concentrated total RNA from up to 25 mg of tissue. Purified RNA for up to 16 samples is obtained in less than 45 min of hands-free instrument operation. No post-purification treatment with nuclease, cleanup, or concentration is required to achieve superior performance in downstream applications. The low elution volume (30–100 μL) is used to generate purified RNA in a more concentrated format for use in downstream applications such as qRT-PCR, RT-PCR, and complementary DNA (cDNA) synthesis.
The integrity and purity of total RNA were determined by a spectrophotometer NanoDrop ™ (Thermo Scientific), measuring the absorbance at 260 nm and the absorbance ratio 260/280, respectively. The integrity of RNA was verified by electrophoresis on 1% agarose gel stained with ethidium bromide.
Reverse transcription of 1 μg total RNA from each fish intestine tissue was performed with random decamers in a volume of 20 μL by using the High-Capacity cDNA Archive Kit (ThermoFisher Scientific, Milan, Italy) following the manufacturer’s instructions.
The number of transcript copies of target genes IL-1β (GenBank acc. nr. AJ269472), IL-6 (GenBank AM490062), IL-8 (AM490063), IL-10 (AM268529), and TNFα (DQ070246) was quantified by One-Step Taqman® real-time RT-PCR technique using the standard curve method. Standard curves were constructed using the known copy number of synthetic mRNAs of each gene. To obtain synthetic mRNAs, a forward and a reverse primer were designed based on the mRNA sequence of each gene and used to create templates for the in vitro transcription of mRNAs (Table 2).
The forward primer was engineered to contain a T3 phage polymerase promoter gene sequence to its 5′ end and used together with the reverse primer in a conventional RT-PCR of total RNA extracted from the sea bass intestine.
PCR products were then evaluated on a 2.5% agarose gel, cloned using pGEM®-T Easy cloning vector system (Promega, Milan, Italy), and subsequently sequenced. In vitro transcriptions were performed using T3 RNA polymerase and other reagents supplied in the Promega RiboProbe In Vitro Transcription System kit according to the manufacturer’s protocol.
The mRNAs produced by in vitro transcription were used as quantitative standards in analyzing experimental (biological) samples (Terova et al. 2009). Defined amounts of mRNAs at tenfold dilutions were subjected to real-time PCR using One-Step Taqman® EZ RT-PCR Core Reagents (ThermoFisher Scientific, Milan, Italy), including 1× Taqman® buffer, 3 mM Mn(OAc)2, 0.3 mM dNTP except dTTP, 0.6 mM dUTP, 0.3 μM forward primer, 0.3 μM reverse primer, 0.2 μM FAM-6 (6-carboxyfluorescein-labeled probe), 5 units rTH DNA polymerase, and 0.5 units AmpErase® UNG enzyme in a 25-μL reaction volume. RT-PCR conditions were 2 min at 50 °C, 30 min at 60 °C, and 5 min at 95 °C, followed by 40 cycles consisting of 20 s at 94 °C, and 1 min at 62 °C. The Ct values obtained by amplification were used to create standard curves for target genes.
One hundred nanograms of total RNA extracted from the experimental intestines was subjected to One-step Taqman® quantitative real-time RT-PCR, in parallel to tenfold-diluted defined amounts of standard mRNA, under the same experimental conditions as used to establish the standard curves. Real-time Assays-by-Design SM PCR primers and gene-specific fluorogenic probes were designed by Life Technologies. Primer sequences and TaqMan® probe used for each target gene amplification are shown in Table 3. TaqMan® PCR reactions were performed on a StepOne Real-Time PCR System (Life Technologies). To reduce pipetting errors, master mixes were prepared to set up duplicate reactions (2 × 30 μL) for each sample.
Data from the TaqMan® PCR runs were collected with StepOne™ Software v 2.0. Cycle threshold (Ct) values corresponded to the number of cycles at which the fluorescence emission monitored in real time exceeded the threshold limit. The Ct values were used to create standard curves to serve as a basis for calculating the absolute amounts of mRNA in total RNA.
All statistical analysis were performed by using the software IBM SPSS Statistics 21. Growth performance data were submitted to two-way analysis of variance (two-way ANOVA) considering both tank and diet effects. One-way ANOVA was applied to analyze the morphology and gene expression data. In all statistical analyses, each individual fish was considered as an experimental unit. Duncan’s test was used for post hoc analysis. Differences were considered to be statistically significant when P < 0.05.
Results
As reported in Table 4, statistical analysis by two-way ANOVA showed significant differences in fish growth performance parameters (SGR, and FCR) related to diet, whereas no tank effect was observed. Growth performances of fish fed for 60 days with diet T were significantly higher (P < 0.05) than in fish fed with diets C and B, whereas there were no significant differences between fish fed diet C and diet B. The condition factor (K) of fish fed with diet T was significantly higher (P < 0.05) than in fish fed with diet B, whereas there were no significant differences between fish fed diets C and T and diets C and B.
Fish were fed for 60 days with three experimental diets (C, B, and T), which contained the same low percentage of fishmeal and high percentage of soybean meal. Diets B and T were supplemented with 0.2% of sodium butyrate or taurine, respectively, whereas Diet C (control), was not supplemented. Values are mean ± SD (n = 10). Statistical differences were calculated by two-way ANOVA followed by Duncan's post hoc test. The values in each row with different superscript letters are significantly different (P < 0.05)
By light microscopy, we evaluated different aspects of the various anatomical portions of the distal intestine: the thickness of muscle and mucosal layers and the shape and length of the villi of the intestinal mucosa. In addition, we paid attention to the presence and distribution of Goblet cells and to the presence, size, and content of supranuclear vacuoles of enterocytes. The presence and extent of any inflammatory infiltrates were also examined.
In the distal intestines of sea bass fed with diet C, the thickness of the intestinal wall was formed by 48% of muscle and 52% of villi (Fig. 1a). In enterocytes, we observed abundant vacuoles of various sizes not regularly aligned, eosinophils and PAS-positive, occupying much of the cytoplasm and forcing the nucleus at the base of the cell (Fig. 1b, c). We observed a few Alcian blue positive Goblet cells that were uniformly distributed (Fig. 1c). An important leukocyte infiltrate CD45+ was found in the lamina propria, at the level of the submucosa and also at the level of the muscle layers (Fig. 1d).
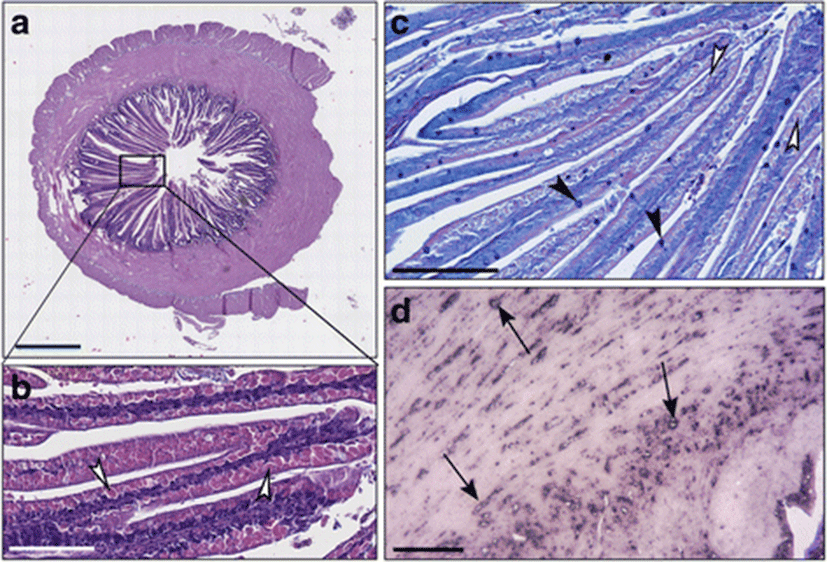
In the intestines of sea bass receiving the diet B in which 0.2% of butyrate was added, the thickness of muscular layer in the intestinal wall was significantly reduced in comparison to fish fed on diet C, whereas the villi layer was elongated and irregularly shaped (Fig. 2a, b; Fig. 3). The intestinal villi were long and branched (Fig. 2a, b). Supranuclear vacuoles diverse in form, size, and content were observed (Fig. 2b). The enterocytes vacuoles were PAS-negative while numerous Goblet cells were found well distributed along the villi, as demonstrated specifically by Alcian blue staining (Fig. 2c). In several areas, the typical single layer showed an abnormal pseudostratification (Fig. 2c). An inflammatory infiltrate CD45+ was identified by immunohistochemistry in the lamina propria and in the submucosa (Fig. 2d).
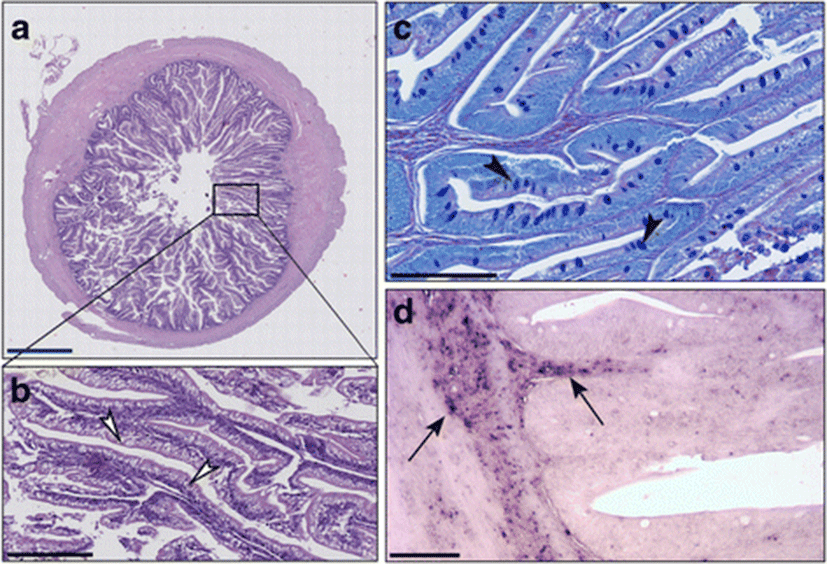
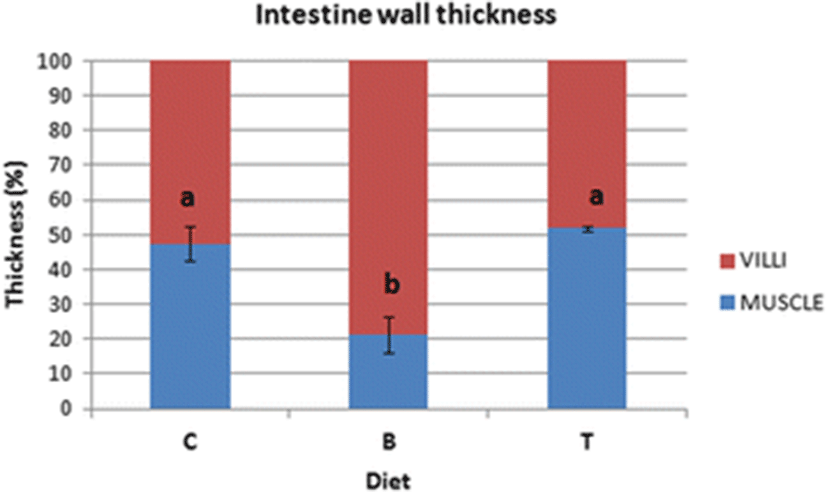
In the sea bass fed with diet T which was supplemented with 0.2% of taurine, the muscle layer and intestinal mucosa had a 1:1 ratio (Figs. 3 and 4a). Supranuclear vacuoles, characterized by a heterogeneous content, were evident (Fig. 4b). Alcian blue positive Goblet cells were abundant, preferentially concentrated in the medium-apical villi, while vacuoles were faintly PAS+ (Fig. 4c). The CD45+ cell infiltrate was detected both in the lamina propria and in the submucosa, as confirmed by immunohistochemistry (Fig. 4d).
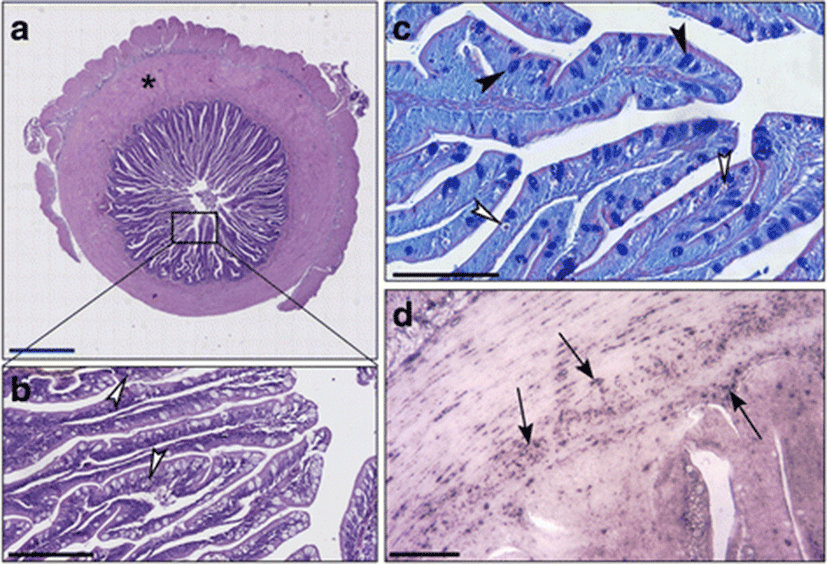
Further investigation by using transmission electron microscopy allowed us to highlight the cell differences between the three types of samples. Different aspects of the enterocytes were investigated. From the lumen to the lamina propria of the fold, we considered the brush border, the tubulo-vesicular system occupying the apical area of enterocytes, the supranuclear vacuoles, the nuclei, and infiltrating inflammatory cells.
The distal intestines of sea bass receiving diet C showed brush border areas with microvilli that appeared irregular and damaged (Fig. 5a). The supranuclear cytoplasm was occupied by numerous vacuoles of different dimensions, with an electrondense content from homogenous to granular (Fig. 5a, b). Some vacuoles showed an irregular contour, suggesting a rupture of their membrane (Fig. 5a). Images of fusion between vacuoles were observed (Fig. 5b). Some nuclei of enterocytes appeared pyknotic (Fig. 5c). An infiltrate of leukocytes was shown between epithelial cells (Fig. 5a, c).
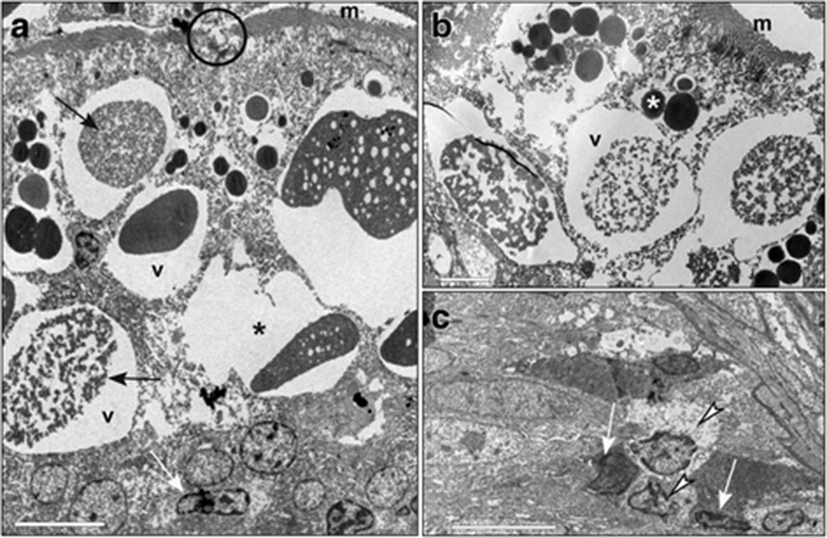
In the intestines of fish fed with diet B, the microvilli were well developed (Fig. 6a, c), even if sometimes interrupted for alteration of the apical plasma membrane (Fig. 6a). In the apical cytoplasm, an expanded tubulo-vesicular system was observed (Fig. 6c). In the supranuclear cytoplasm, many characteristic vacuoles that had an irregular shape and heterogeneous content, with clear areas mixed with dense material, were evident (Fig. 6b, c). Between enterocytes some Goblet cells were present (Fig. 6a).
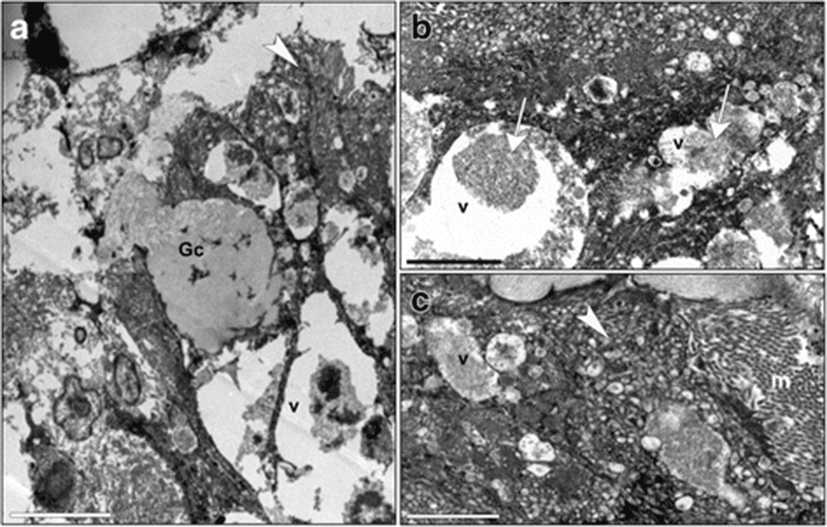
Sections of distal intestines from fish fed with diet T presented microvilli, which were long and well-shaped, sometimes topped by a layer of mucus and a tubulo-vesicular system in the apical cytoplasm (Fig. 7a, c). In the supranuclear cytoplasm, there were many vacuoles with a heterogeneous content showing some lamellar profiles (Fig. 7b). Some Goblet cells were observed between enterocytes (Fig. 7a).
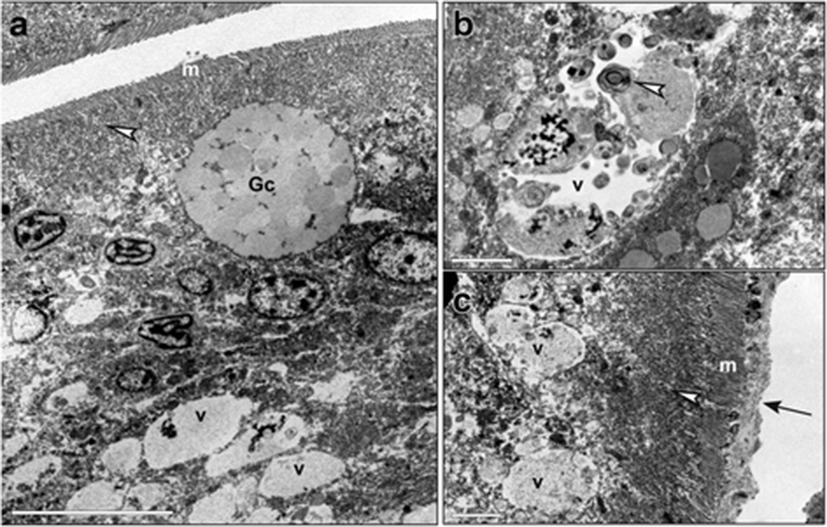
The expression levels of the proinflammatory interleukins (IL-1β, IL-6, IL-8), TNFα and the anti-inflammatory interleukin IL-10 genes were evaluated in the distal portion of sea bass intestine by One-Step Taqman® real-time RT-PCR. The results of this analysis (Table 5) showed that the expression of target genes in the intestine was deeply influenced by the type of fish diet.
The number of transcript copies of target genes was quantified by One-Step Taqman® real-time RT-PCR technique using the standard curve method. Values are mean ± SD (n = 6). The values in each row with different superscript letters are significantly different (P < 0.05)
Although IL-1β was expressed at relatively low levels in all analyzed fish, sea bass fed with diet T (supplemented with taurine), displayed the lowest number of mRNA copies of IL-1β gene in comparison to fish fed with the other two diets. Also, the expression of IL-8 and IL-10 genes in fish fed diet T was significantly lower (P < 0.05) with respect to the fish that received control or butyrate-supplemented diets. Dietary butyrate caused an upregulation of TNFα gene transcription (Table 5); indeed, fish fed on diet B showed higher levels of TNFα mRNA copies than fish fed with the other diets (P < 0.05). Finally, among the interleukins quantified in this study, IL-6 was the only one, to be not influenced by the diet (Table 5).
Discussion
The feeding experiment conducted in this study lasted 60 days which is a short period for SGR and FCR reliable evaluation. Nevertheless, SGR of fish fed with diet T was 2.7 times higher (P < 0.05) than in fish fed with diet C or B. This result is also in line with findings of Brotons Martinez et al. (2004) on sea bass fingerlings fed on a diet containing SBM and supplemented with taurine for 30 days. The dramatic differences in growth observed in our study between the taurine-supplemented group (T) and the experimental groups B and C may be due to taurine deficiency in the latter groups, thus indicating that taurine is an essential dietary nutrient, as reported in several studies reviewed by Salze and Davis (2015).
Histological samples from distal intestine of fish fed with diet B (supplemented with sodium butyrate) showed that the muscle layers were thinner than in fish fed with diets C or T. This suggests that butyrate facilitated the formation of softer fecal pellets in fish of this group. Furthermore, the complex structure of the intestinal villi included a high number of Goblet cells, and it was not pseudostratified which means that it was iperproliferative. This result is in line with previous studies (Gálfi and Bokori 1990; Kotunia et al. 2004; Hu and Guo 2007), which have demonstrated the trophic effects of butyrate on the intestinal epithelium of terrestrial animals through an increase in the villi length and crypt depth. The number of supranuclear vacuoles was less than in fish fed with diet C, and they were often heterogeneous. We observed a few intestinal Goblet cells, in fish fed with diet C with SBM, and this result is in agreement with other studies conducted in Atlantic salmon (S. salar) and rainbow trout (O. mykiss) (Baeverfjord and Krogdahl 1996; Iwashita et al. 2008). However, we found also high concentrations of absorptive vacuoles containing PAS-positive substances (probably glicoproteins, considering their acidophilic properties) in the supranuclear region of the enterocytes from fish fed with diet C. This could indicate an interruption of the digestive processes occurring within the enterocytes, since these cells are involved not only in the uptake of different nutrients but also in the digestion. For example, once inside the enterocyte, the absorbed di- and tripeptides are digested into amino acids by cytoplasmic peptidases and transported from the cell into blood; only a very small number of these small peptides enter blood intact. In contrast, other authors have reported a reduction of the same supranuclear vesicles in salmonids and in carps. In fish fed with diet C, lamina propria, muscle layer, and epithelial lining were heavily infiltrated with inflammatory cells, including macrophages and polymorphonuclear leukocytes. The important signs of inflammation in our samples were also confirmed by immunohistochemistry staining. This analysis showed a strong positivity for anti-CD45 antibody indicating a relevant diffusion of leukocytes in all the distal intestinal tissue. The fact that an antibody directed against the human protein reacted also with sea bass tissue might confirm a high structural conservation of this protein in fish as well. This hypothesis is also supported by the discovery of a CD45 homolog (Marozzi et al. 2012) in sea bass (D. labrax), whose sequence obtained from transcriptome of gills (kindly provided by Francesco Buonocore, University of Tuscia, Italy) shows 52% of identity with human CD45 isoform 1 precursor. Since a common rule of thumb is that two sequences are homologous if they share more than 30% of identity over their entire length (Pearson, 2013), we are confident that 52% is a high enough identity to support the similarity between human and sea bass CD45 sequences. Of note, a 30% identity threshold for homology underestimates the number of homologs detected by sequence similarity between humans and yeast by 33% (Pearson 2013).
The TEM observations showed partly damaged microvilli and numerous large vacuoles occupying the supranuclear cytoplasm and containing a homogeneous electrondense substance, with partial break of their membranes probably leading to the release of lytic enzymes and recall of leukocytes. Some enterocytes with pyknotic nuclei were also observed. According to Noaillac-Depeyre and Gas (1973), the supranuclear body is of complex origin. Indeed, in the adult carp, the apical cytoplasm of the enterocytes of the medium intestine shows the presence of a dense tubolovesicular network that form a voluminous supranuclear body. However, the supranuclear bodies are not related to the presence of food in the intestinal lumen since they are also present in fasting animals. Therefore, it seems that they do not result only from the running together of the food vacuoles but probably arise from the fusion of the Golgi vesicles. The supranuclear bodies would thus have a dual origin, endogenous for the hydrolytic enzymes that contain, and exogenous for the di- and tripeptides supplied by the food which are then further digested inside them (Noaillac-Depeyre and Gas 1973).
Furthermore, the aforementioned acidophilic and PAS-positive substance that we found in the vacuoles of fish fed with diet C was absent in the distal intestine of fish fed with diet B, suggesting that butyrate supplementation of the diet have had a mitigation effect in the intestine of fish.
Although the inflammatory cells were infiltrated less than in the distal intestine of fish fed with diet C and were more concentrated at the mucosal and submucosa level, we found a significant number of leukocytes as confirmed by the positive staining with the CD45 antibody. TEM analysis of enterocytes showed a focal alteration at the microvilli level. However, other areas appeared much less compromised, microvilli were regularly displayed, and a developed apical tubulo-vesicular system was evident, suggesting an intense phagocitosys activity The cells showed supranuclear vacuoles with dishomogeneous content: partly dense, like that found in the fish of group C, and partly clear; this aspect suggests that in these vacuoles a partial digestion of the content occurs.
In fish fed with diet T, the muscle layers were rather developed. The villi folds were long and regular, with enterocytes showing a limited number of vacuoles and an increased number of Goblet cells confirming a strong inflammation reaction, which were mainly concentrated in the medioapical part of the fold. Leukocytes, immunoreactive to the CD45 antibody, were still present in both, the mucosa and the submucosa, similar to those observed in fish fed with diet B. The TEM images of enterocytes were very different from those in fish fed with diets C and B, with cells showing well-displayed microvilli frequently covered by a protective mucus layer, no alterations in the structure, an apical tubulo-vesicular system, and supranuclear vacuoles filled with a heterogeneous substance, including membranous bodies suggesting that an autophagic process was in progress. To our knowledge, no other example of taurine used as a feed additive causing autophagic processes has been reported in the literature, and thus this finding should be further investigated. However, this process seems to enable enterocytes of fish fed with diet T to cope with the negative effects induced by the SBM diet and contributes to the protection of the intestinal mucosa.
The expression pattern of molecular markers of inflammatory processes such as the inflammatory cytokines supported the light and TEM microscopy observations. The highest transcription level of the pro-inflammatory IL-1β gene was found in the distal intestine of sea bass fed with diet C, indicating a local tissue reaction to SBM insult, which was less evident in fish fed on diets B or T. Cytokine IL-8 was less expressed in fish fed with diet T than in those fed with diet C or B, suggesting a protective action of taurine against oxidative stress through the reduction of the inflammatory cascade induced by oxidative stress. The transcription activity of IL-10 gene, which is known as anti-inflammatory cytokine, was strongly reduced in fish fed with diet T, remaining ,however, relatively high in fish fed with diets C and B. Among the target cytokines, only IL-6 gene expression was not affected by dietary butyrate or taurine supplementation. Proinflammatory IL-6 is of particular interest since it is expressed to a small extent in normal colonic epithelium but to a much greater degree in colonic carcinomas (Shirota et al. 1990). In human colonic cells, increased expression of both IL-6 and its receptor IL-6R have mainly an anti-apoptotic effect (Yuan et al. 2004). IL-6, indeed, upregulates the expression of anti-apoptotic protein Bcl-xl (Fujio et al. 1997; Scwarze and Hawly 1995). Yuan and colleagues (2004), clearly demonstrated that butyrate downregulates IL-6 signaling in human colonocytes by inhibiting IL-6 receptor rather than IL-6 expression. Similarly, in our study, butyrate did not influence IL-6 expression, but we cannot exclude that, even in fish, butyrate could act principally on IL-6 receptor rather than in IL-6 expression. So, this is an issue that requires further investigation.
It is well documented that taurine inhibits the overproduction of inflammatory mediators such as TNFα (Kim and Cha 2014; Locksley et al. 2001). TNFα is known to stimulate further production of itself and to induce the expression of other pro-inflammatory mediators, such as IL-6, IL-8. TNFα was expressed at higher levels in the intestine of sea bass fed with diet B, which induced an upregulation also of IL-8 but not of IL-6. Moreover, higher expression of TNFα in fish receiving B diet supported the TEM results indicating a promotive effect of butyrate in increasing cellular turnover.
Our findings on cytokine activity are mainly in agreement with the literature. Indeed, in the intestine of carps receiving soy as protein source (Urán et al. 2008a), a peak of IL-1β was observed after the first week of feeding, and the expression of this gene as well as that of TNFα remained high over the control levels for the entire experiment. In the same study, a strong upregulation of IL-10 was observed after one week of SBM feeding, but at weeks 3 and 5, expression levels were downregulated at values either lower or similar to the control (Urán et al. 2008a). In zebrafish fed on diets containing different amounts of SBM, Hedrera et al. (2013) reported a significant increase in the intestinal transcription of the mRNA coding for proinflammatory cytokines, such as IL-1β and IL-8. The transcriptional activity of IL-10, known to have an anti-inflammatory action, was increased in the group receiving feed with soy protein, too.
Conclusions
European sea bass fed on a diet containing a concentration of a soy protein source close to 30% (16.7% as SBM and 12.8 as full-fat soy) for 2 months developed an inflammatory status in the distal intestine. Both butyrate and taurine, at concentrations as low as 0.2%, had some mitigating effect on the symptoms of inflammation, but the underling mechanisms of action seem different. The mechanisms underlying these effects should be further investigated along with any possible synergistic effect of butyrate and taurine in the feed in normalizing the intestinal abnormalities caused by soybean meal.