Introduction
Acetylcholine (ACh) is the major neurotransmitter synthesized from acetyl CoA and choline, by choline acetyltransferase (ChAT) in the cytoplasm (Ferreira-Vieira et al., 2016). It is uptaken by the vesicular acetylcholine transporter (VAChT) into the synaptic vesicles to convey a synaptic signal to the postsynaptic neurons through its binding to nicotinic ACh receptors (nAChR) or muscarinic ACh receptors (mAChR) (Silverthorn et al., 2016). In the synaptic cleft, ACh is promptly hydrolyzed to acetate and choline through the catalysis of acetylcholinesterase (AChE) enzyme and recycled into the presynaptic neuron (Colovic et al., 2013). Alzheimer’s disease damages the brain cells that produce or use ACh, thereby reducing the amount of neurotransmitters available to carry neurological signals; whereas the amount of ChAT, nAChR, and mAChR are also abnormally downregulated in Alzheimer’s patients (Kihara & Shimohama, 2004; Lombardo & Maskos, 2015). Furthermore, the natural process of ACh metabolism facilitated by AChE further reduces the amount of ACh and weakens the transportation of electrical impulses in the cholinergic system (Hung & Fu, 2017). This results in impairments in motor function, memory and attention in patients.
While there is currently no cure for Alzheimer’s, clinicians have applied cholinesterases inhibitors (ChEIs) to prevent the hydrolysis of ACh by cholinesterases (ChEs) (Hung & Fu, 2017). The drugs indirectly aid in maintaining the ACh level that is important for learning and memory, and compensating the loss of functioning brain cells; thus, they enhance the symptoms related to memory thinking, language, judgment and other cognitive processes (Alzheimer’s Association Report, 2021). To date, three out of five U.S. Food and Drug Administration (FDA) approved symptomatic medications for Alzheimer’s disease are ChEIs, namely Aricept (donepezil), Exelon (rivastigmine), and Razdyne (galantamine) (Alzheimer’s Association Report, 2021). Certain ChEIs seems to offer other benefits, for example, galantamine appears to stimulate the release of ACh and improve the way certain message-receiving neurons respond to it (Alzheimer’s Association Report, 2021); while rivastigmine is able to intensify the cholinergic activity by providing dual inhibition of AChE and butyrylcholinesterase (BuChE), which further extend its usage in subcortical vascular and Parkinson’s disease dementia (Kandiah et al., 2017). Besides, Donepezil was reported with several beneficial impacts on behavioral and neuropsychiatric symptoms, such as hallucinations, distractibility, aberrant motor behavior and apathy (Tsuno, 2009). Despite a small degree of improvement, clinical trials of all ChEIs are overall showing enhanced memory and thinking compared to placebo, as well as delay or slow worsening of Alzheimer’s disease symptoms in up to 40% of individuals who taken the medication (Mielke et al., 2012). Despite all the benefits above, ChEIs do not reverse Alzheimer’s nor stop the underlying degeneration of nerve cells. In fact, there is still risk of side effects that are unavoidable such as nausea, vomiting, gastrointestinal bleeding, bronchospasm and skin patches (Ruangritchankul et al., 2021). Knowing that nearly 10 million dementia patients are increasing every year (World Health Organisation, 2021) and the effect of current drugs are still in a large space to be improved, growing demand of getting better ChEIs with greater effect and lower side effects are gradually catching up, which has led us to explore any possibilities from the novel resources such as marine algae.
Consumption of algae has been revealed to prevent neurodegeneration. Studies showed that a higher seaweed intake in the Japanese diet and other East Asia countries compared to the European countries poised a lower neurodegenerative disorder risk (Okubo et al., 2017; Pangestuti & Kim, 2011). In Malaysia, red seaweed Gracilaria is commonly used in traditional dishes for the Malay natives along the east coast of Peninsular Malaysia as Kelantanese seaweed salad named ‘Kerabu Sare’. Gracilaria species including Gracilaria changii, Gracilaria firma, and G. manilaensis Yamamoto & Trono are potential agarophytic species that provide raw materials for agar production in the food industry and as a food source for shellfish and shrimp (Ng et al., 2017; Phang et al., 2019; Yow et al., 2013). Current findings on G. manilaensis showed that it possesses various bioactive compounds with potential therapeutic effects. This includes hexadecanoic acid and 2-hydroxymyristic acid that portray anticancer property, 1-(4’-methoxyphenyl)-3-(2,4,6-trihydroxyphenyl)-3-hydroxypropanone that portrays antioxidant property, as well as cholesteryl myristate that portrays antibacterial property (Andriani et al., 2016). G. manilaensis from Malaysia also possesses the neuritogenic effect and in vitro antioxidant activities (Pang et al., 2018). The proven neurological benefits have driven our interest to further investigate the anti-AChE activity of G. manilaensis and to determine the bioactive substance(s) that is responsible for this activity.
Materials and Methods
Fresh specimens of G. manilaensis were collected from Kuala Muda, Kedah. The specimens were washed with salt water to remove sands, mud and impurities, followed by the final rinse in distilled water and later froze. The frozen specimens were then freeze dried (Labogene, Germany) and pulverised. G. manilaensis powder was kept at −20°C prior to extracts preparation.
G. manilaensis extracts were prepared from the powder by maceration as described in Pang et al. (2018). Briefly, 1 g of seaweed powder was dissolved into hot boiling water, methanol and ethanol at the ratio of 1:50 (w/v) respectively. For hot aqueous extract (GMHW), the mixture was incubated in roller mixer for 30 min at room temperature. The mixture was centrifuged at 2,688×g for 20 min at 4°C. After that, the supernatant was frozen and concentrated with a vacuum concentrator (Labogene, Germany). The dried product, GMHW was stored at −20°C until used. Meanwhile, for methanol and ethanol extracts (GMM and GME), the solvent-powder mixtures were incubated in an incubator shaker at 9×g for 36–48 h at room temperature. The mixture was centrifuged at 2,688×g for 20 min at 4°C. Subsequently, the supernatants were further concentrated using a rotary evaporator and vacuum concentrator. Both extracts were stored at −20°C until used.
Ellman assay was used to determine the AChE and BuChE activities using the method described by Yoon et al. (2009) with slight modification. Each reaction was scaled to 200 μL reaction in a 96-well microplate. To prepare GMHW, distilled water was used as the vehicle. For GMM and the positive controls (eserine and isoxazole), 10% aqueous methanol was used as the vehicle. Meanwhile, 10% aqueous ethanol was used to dissolve GME. Briefly, 20 μL of the sample was added into 140 μL of sodium phosphate buffer (pH 8.0) and 20 μL of AChE (0.036 U/mL, C2888, Sigma-Aldrich, St. Louis, MO, USA) or BuChE (0.036 U/mL, C1057, Sigma-Aldrich). After 15 min of incubation, 20 μL of substrate ACh or BuCh (3 mM, Sigma-Aldrich) and 20 μL of 5-5’-thiobis-2-nitrobenzoic acid (DTNB, 5 mM, Sigma-Aldrich) were added into the reaction mixture. The absorbance of each reaction mixture was read at 412 nm at 37°C by the kinetic loop of 3 min intervals for 15 min. The rate of reaction was obtained by the slope of absorbance against the time in minutes. The unit was expressed in absorbance/min. The AChE or BuChE inhibitory activity (%) was obtained by the formula:
The half-maximum effective concentration (EC50) values of the positive controls and the extracts were measured by adapting from the dose-depending relationship curve of AChE or BuChE activity % against the concentration of respective treatment. Three independent experiments were carried out to obtain the data in mean ± SD.
Enzyme kinetic study was also carried out using the method described by Yoon et al. (2009) with slight modification. The concentrations of GMM and GMMG were fixed at their respective EC25 or EC50. Substrates of ascending concentration from 3, 6, 9, 12, and 15 mM were added after 15 min of incubation. The reciprocal value of the rate of reaction (1/V) and the reciprocal value of the substrate concentration (1/[S] mM) was used to plot the line-weaver Burk plot. The values of enzyme activity (Km, mM), the maximum rate of reaction (Vmax, Absorbance/min) and inhibition constant (Ki, mg/mM) were obtained by three independent experiments. These experiments were repeated for its quarter-maximum EC25.
GMM was selected as the most potent extract to be further investigated in this study. To produce fractions, 200 g powder was extracted with 2 L of absolute methanol for five times. After the removal of powder by filtration, approximate 10 g of GMM was concentrated with a rotary evaporator, and then dissolved in absolute chloroform. The GMM (10 g) was subjected to column chromatography over silica gel 60, elution successively with hexane-chloroform (4:6–1:9), chloroform, chloroform-methanol (99:1–90:1) and ethanol to produce 10 main fractions based on thin-layer chromatography (TLC) profile: GMMA (75.1 mg), GMMB (46.6 mg), GMMC (41.9 mg), GMMD (129.6 mg), GMME (97.6 mg), GMMF (283.4 mg), GMMG (272.6 mg), GMMH (85.6 mg), GMMI (339.2 mg), and GMMJ (1,227.3 mg).
GMMG was subjected to LCMS analysis with Agilent 1290 Infinity LC system coupled to Agilent 6520 Accurate-Mass Q-TOF mass spectrometer with dual ESI source (Agilent Technologies, Santa Clara, CA, USA). The column used was Agilent Zorbax Eclipse XDB-C18, Narrow-Bore 2.1 × 150 mm, 3.5 micron (P/N: 930990-902) with mobile phase 0.1% formic acid in water and 0.1% formic acid in acetonitrile. 1 μL of sample (1 mg/mL) was injected at the flow rate of 0.5 mL/min. The result of chromatogram data was analysed with Agilent MassHunter Qualitative Analysis B.07.00 (Method: Metabolomic-2019.m) matched with Metiln database based on mass over charge (m/z). Compounds were shortlisted by elimination of blank, include all the compounds with Score Db or Score MFG ≥ 90, and with Difference (Db.ppm) or Difference (MFG.ppm) that is ± 5. Activities of the known compound were matched with publication and database on Web of Science and PubChem.
Results
All G. manilaensis extracts possessed anti-AChE activity in majority of the tested concentrations (Fig. 1). Meanwhile, none of the extracts but isoxazole showed significant anti-BuChE activity (p < 0.05) (Fig. 2). GMHW produced significant anti-AChE activity of 10.98 ± 1.67% at concentration as low as 0.0625 mg/mL (p < 0.005). The highest activity of GMHW observed was 38.23 ± 1.05% at 4 mg/mL. On the other hand, GMM and GME only showed significant anti-AChE activity at 0.125 mg/mL, with 8.62 ± 3.73% and 15.21 ± 1.28% respectively (p < 0.01 and p < 0.005). However, anti-AChE activity of GMM and GME observed a rapid increment from 2 mg/mL to 4 mg/mL, which resulted in a higher anti-AChE activity of 69.3 ± 7.16% and 49.94 ± 0.23% at 4 mg/mL respectively. Since GMM was the only extract that showed anti-AChE activity higher than 50% at 4 mg/mL, it was selected for the downstream fractionation process.
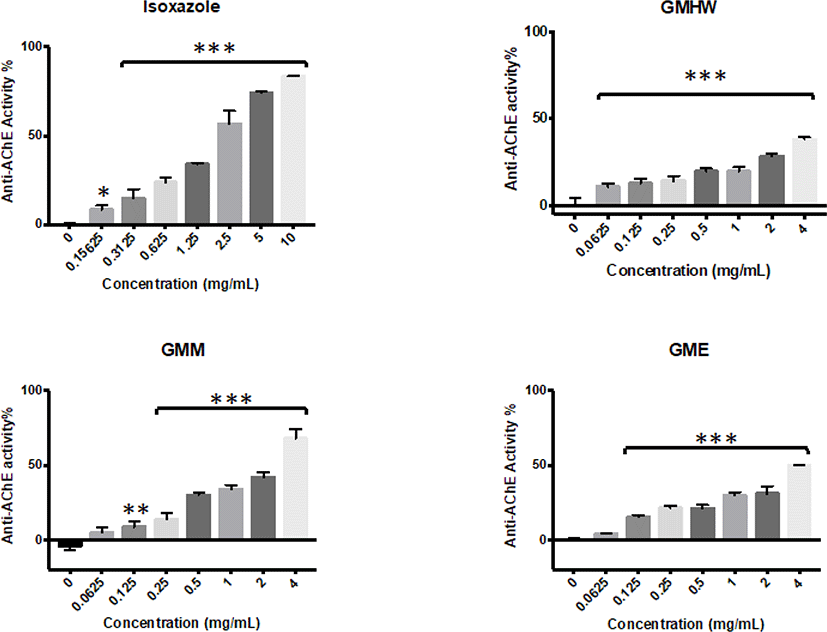
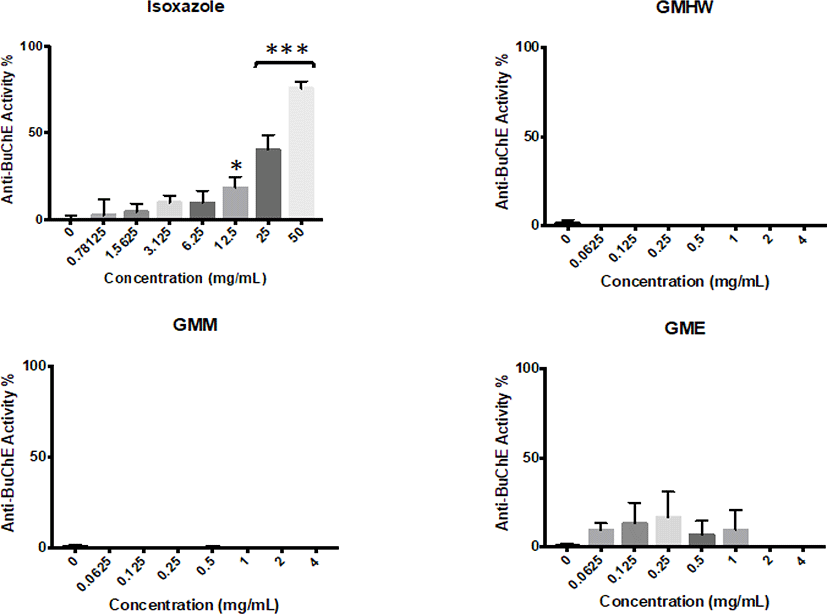
GMM was fractionated into 10 fractions according to the TLC profile (Fig. 3). All fractions except GMMD possessed significant anti-AChE activity (p < 0.05) against the negative control (Fig. 4). GMMA and GMME showed the lowest anti-AChE activity at 33.8 ± 4.6% and 37.1 ± 3.8% respectively. Meanwhile, GMMF, GMMH, and GMMJ showed moderate activity at 43.4 ± 7.7%, 45.3 ± 1.5% and 41.7 ± 1.9% respectively. Furthermore, GMMB, GMMC, and GMMI showed moderate-high anti-AChE activity at 54.4 ± 4.8%, 62.0 ± 1.1 and 53.6 ± 1.5% respectively. GMMG showed the highest anti-AChE activity at 69.2 ± 5.1%; thus, GMMG was selected for subsequent assay of cholinesterase inhibitory activities.
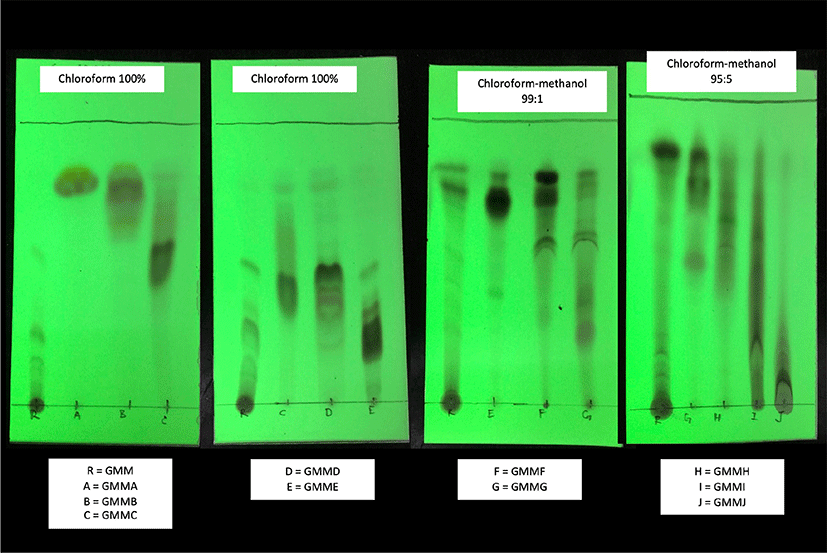
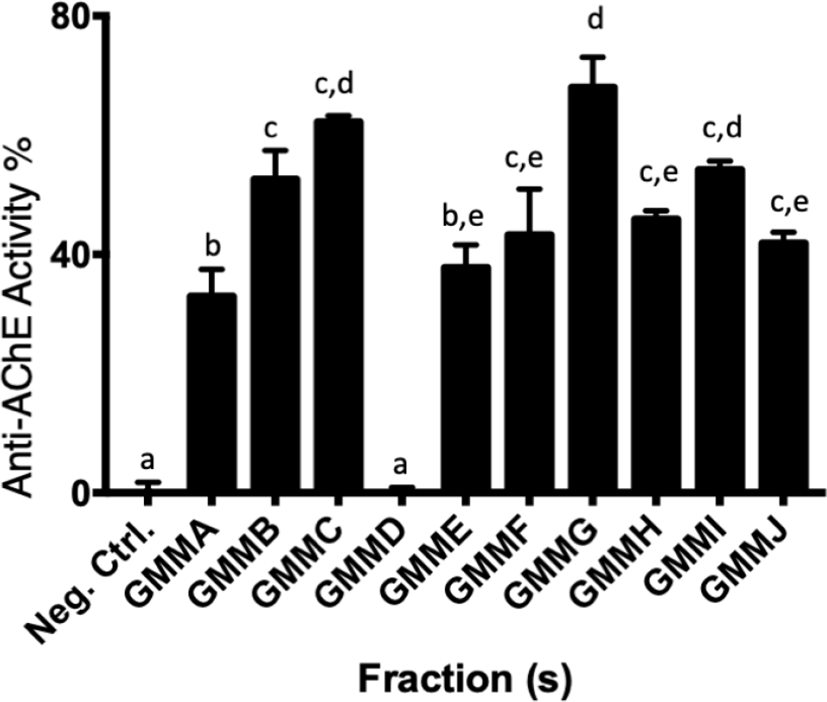
GMMG showed positive AChE activity but negative BuChE activity (Fig. 5), similar to its predecessor, GMM. At the concentration of 0.375 mg/mL, GMMG started to show significant anti-AChE activity with 20.89 ± 0.27%. The anti-AChE activity of GMMG increased with the concentration, where it reached the highest at 3 mg/mL with 61.09 ± 6.15%.
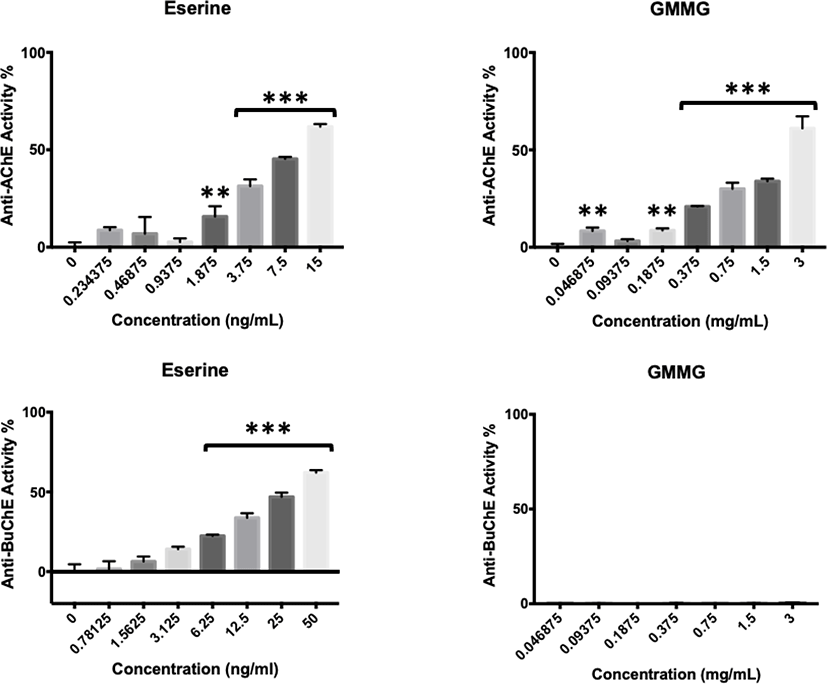
Anti-AChE and BuChE activities of G. manilaensis and the positive controls (eserine and isoxazole) expressed in EC50 (Table 1). In this study, eserine and isoxazole were used as the positive control. Eserine has been optimised in vitro in the Ellman assay as described in Yoon et al. (2008, 2009), whereby isoxazole is a novel AChE inhibitor described in Gutiérrez et al. (2013) which is effective against AChE from Electroporus electricus, the similar AChE used in our study model (C2888, Sigma-Aldrich). Notable, eserine showed the lowest EC50 at 10.6 ± 2.0 ng/mL, whereas GMM and GMMG showed anti-AChE activity with EC50 at 2.60 ± 0.16 mg/mL and 2.22 ± 0.27 mg/mL respectively, significantly lower than isoxazole and GME (p < 0.05). For BuChE, all samples were negative within the tested range, hence no EC50 value was shown. Based on Andriani et al. (2016), methanol was the best solvent to extract the anti-AChE compound(s) from G. manilaensis, which was similar to our findings. GMM and GMMG warrant a high potential for further testing.
GMM and GMMG were tested for their enzyme inhibition kinetic using the Lineweaver-Burk plot (Figs. 6 and 7). The presence of GMM increased the value of Km from 1.48 mM to 1.68 mM at EC25 (1.3 mg/mL) and 2.03 mM at EC50 (2.6 mg/mL). However, the value of Vmax was decreased from 0.0281 mM to 0.0231 mM at EC25 (1.3 mg/mL) and 0.0213 mM at EC50 (2.6 mg/mL) by GMM (Table 2). This matched the mixed-inhibition pattern of the Lineweaver-Burk plot, suggested that the GMM might possess bioactive compound(s) which can bind with both the enzyme and enzyme-substrate complex, with a higher affinity to the enzyme. GMM could be a mixed inhibitor to the anti-AChE activity.
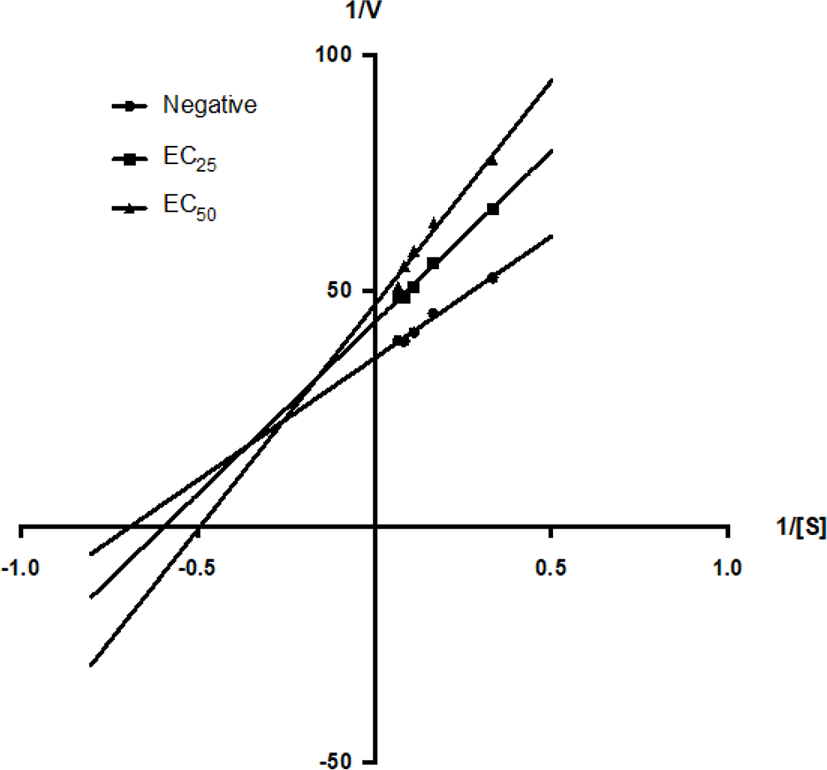
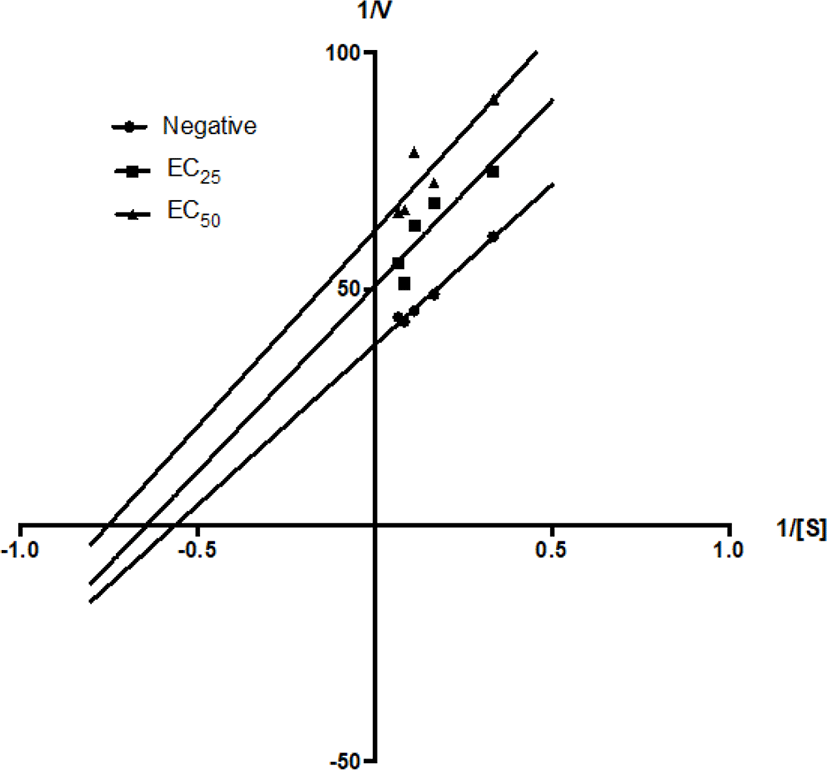
Inhibitors | Km (mM) | Vmax (mM) | Ki |
---|---|---|---|
Without inhibitor | 1.48 | 0.028 | |
EC25 (1.3 mg/mL) | 1.68 | 0.023 | 3.36 |
EC50 (2.6 mg/mL) | 2.03 | 0.021 | 3.18 |
On the other hand, GMMG decreased the value of Km from 1.78 mM to 1.55 mM at EC25 (1.15 mg/mL) and 1.33 mM at EC50 (2.30 mg/mL). The value of Vmax was decreased from 0.026 mM to 0.02 mM at EC25 (1.15 mg/mL) and 0.016 mM at EC50 (2.30 mg/mL) by GMMG (Table 3). This matched the uncompetitive inhibition pattern of the Lineweaver-Burk plot, suggested that the GMMG possess bioactive compound(s) which can bind with the enzyme-substrate complex specifically. GMMG could be an uncompetitive inhibitor to the anti-AChE activity.
Inhibitor | Km (mM) | Vmax (mM) | Ki |
---|---|---|---|
Without inhibitor | 1.78 | 0.026 | |
EC25 (1.15 mg/mL) | 1.55 | 0.020 | 7.21 |
EC50 (2.30 mg/mL) | 1.33 | 0.016 | 10.32 |
LCMS analysis was carried out and the results detected 136 peaks in the positive ion mass spectra. Of these, 28 peaks were matched with the identity of known molecules on the Metlin database, with Molecular Formula Generator (MFG) scores above 90% and ± 5 differences in MFG scores. The proposed compounds were searched and presented in Table 4. Interestingly, cynerine A, graveolinine, militarinone A, eplerenone and curumenol are the neuroprotective-related compounds detected among the 28 known compounds. In contrast, 10 other compounds that had MFG scores above the cut-off did not match with any molecules in the library, indicating a large family of new unknown compounds that are yet to be explored in GMMG (Table 5). The chromatograms and MS spectra of compounds were shown in Figs. 3 and 8.
No | Name | Formula | m/z | Mass | Group | Activity | Related compound(s) | Activity |
---|---|---|---|---|---|---|---|---|
1 | 4-(2-hydroxypropoxy)-3,5-dimethyl-phenol | C11H16 O3 | 197.12 | 196.11 | Alcohol | • No record | ||
2 | Dioscoretine | C13 H23NO3 | 242.17 | 241.17 | Fatty acid | • No record | ||
3 | 2-Phenylethyl 3-methylbutanoate | C13 H18O2 | 207.14 | 206.13 | Carboxylic acid | • No record | ||
4 | ML-236C | C18H26O3 | 291.20 | 290.19 | Carbobicylic compound | • Anticholesteremic (Endo et al., 1976) | ||
5 | C16 Sphinganine | C16H35NO2 | 274.27 | 273.27 | Lipid: Phospholipid | • Antibacteria (Reid et al., 2019) | ||
• Anticancer (Bagheri et al., 2018) | ||||||||
6 | 2,6-Nonadien-1-ol | C9H16O | 158.15 | 140.12 | Fatty alcohol | • Flavour compound (Kemp et al., 1974; Flath et al., 1983; McGinty et al., 2010; Api et al., 2015, 2019) | ||
7 | (–)-trans-C75 | C14H22O4 | 272.19 | 254.15 | Carboxylic acid | • No record | ||
8 | Cyrneine A* | C20H28O3 | 317.21 | 316.20 | Terpene: Diterpene | • Neurite outgrowth (Marcotullio et al., 2006; Obara et al., 2007) | Cyathane diterpene group | • Anti-oxidant (Marcotullio et al., 2008) |
• Anti-nitric oxide (Wang et al., 2014) | ||||||||
• Neurite outgrowth (Cao et al., 2018) | ||||||||
• Anti-bacterial (Nitthithanasilp et al., 2018) | ||||||||
9 | 7beta-Hydroxy-lathyrol | C20H30O4 | 335.22 | 334.21 | Lipid: Diterpene | • No record | ||
10 | Graveolinine* | C17H13NO3 | 297.12 | 279.09 | Quinonline | Anti-angiogenesis (An et al., 2010) | ||
Anti-tumor and anti-platelet aggregation (Wu et al., 2003) | ||||||||
Anti-AChE and anti-β amyloid (Luo et al., 2020) | ||||||||
11 | 3α-Hydroxy-4,4-bisnor-8,11,13-podocarpatriene | C15H20O | 217.16 | 216.15 | Phenol | • No record | ||
12 | Militarinone A* | C26H37NO6 | 460.27 | 459.26 | Pyridine alkaloid | • Neurite outgrowth (Schmidt et al., 2002; Riese et al., 2004; Küenzi et al., 2008) | 4-hydroxy-2-pyridone | • Neurite outgrowth (Schröder et al., 2015) |
(+)-N-Deoxymilitarinone A | • Neurite outgrowth (Cheng et al., 2006) | |||||||
13 | Eplerenone* | C24H30O6 | 432.24 | 414.21 | Lactone | • Anti-inflammation (Suzuki et al., 2006; Xiao et al., 2009; Bayorh et al., 2012; Chen et al., 2013, 2018; Łabuzek et al., 2014; Wada et al., 2017; Vecchiola et al., 2020) | ||
• Neuroprotective (Dong et al., 2012; Wang et al., 2020) | ||||||||
14 | Curcumenol* | C15H22O2 | 235.17 | 234.16 | Curcumin | • Anti-neuroinflammation (Tanaka et al., 2008; Lo et al., 2015; Hsiao et al., 2020; Pintatum et al., 2020) | Oxycurcuminol | • Anti-stroke (Chen et al., 2018) |
• Antioxidant | ||||||||
• Neuroprotective (Hamdi et al., 2015) | ||||||||
15 | (+–)-Trihydroxy-decipiadiene | C20H32O3 | 321.24 | 320.23 | Lipid: Isoprenoid | • No record | ||
16 | alhpa-Tocopheronolactone | C16H22O4 | 301.14 | 278.15 | Lipid: Isoprenoid | • No record | ||
17 | N-cis-Tetradec-9Z-enoyl-L-Homoserine lactone | C18H31NO3 | 310.24 | 309.23 | Lactone | • No record | ||
18 | Stigmatellin Y | C29H40O6 | 502.32 | 484.28 | Phenol | • No record | ||
19 | Anandamide (20:l, n-9) | C22H43NO2 | 376.32 | 353.33 | Fatty amide | • No record | ||
20 | 2-Amino-14,16-dimethyloctadecan-3-ol | C20H43NO | 336.32 | 313.33 | Amino alcohol | • No record | ||
21 | 2-Ethyl-dodecanoic acid | C14H28O2 | 229.22 | 228.21 | Fatty acid | • No record | ||
22 | (–)-Isoamijiol | C20H32O2 | 322.27 | 304.24 | Lipid: Isoprenoid | • No record | ||
23 | Palmitic amide | C16H33NO | 256.26 | 255.26 | Fatty amide | • No record | ||
24 | Coproporphyrin IV | C36H38N4O8 | 655.28 | 654.27 | Porphyrin | • No record | ||
25 | Cassaidine | C24H41NO4 | 408.31 | 407.30 | Tricylic diterpenoids | • No record | ||
26 | Haplophytine | C37H40N4O7 | 653.30 | 652.29 | Indole alkaloid | • No record | ||
27 | 24,25-Epoxy-cholesterol | C27H44O2 | 401.34 | 400.33 | Desmosterol | • No record | ||
28 | MGDG(18:1(9Z)/18:1(9Z)) | C45H82O10 | 805.58 | 782.59 | Glycosyldiradylglycerols | • No record |
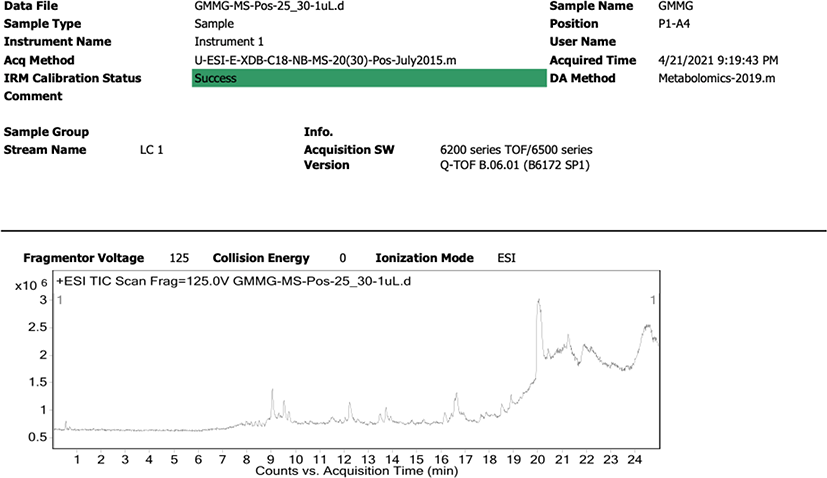
Discussion
To date, there are many red algae with anti-AChE activity have been reported, including Hypnea valentiae (Suganthy et al., 2010), Gracilaria edulis (Suganthy et al., 2010), Gelidiella acerosa (Syad et al., 2012, 2016), Hypnea musciformis (Machado et al., 2015), Ochtodes secundiramea (Machado et al., 2015), Pterocladiella capillacea (Machado et al., 2015), Gelidium foliaceum (Kannan et al., 2013), Amphiroa beauvoisii (Kannan et al., 2013), Laurencia complanate (Kannan et al., 2013), Rhodomelopsis africana (Kannan et al., 2013), Porphyra tenera (Senevirathne et al., 2010), G. changii (Andriani et al., 2016) and G. manilaensis (Andriani et al., 2016). In contrast, this is the first comprehensive study of Malaysian G. manilaensis whereby the anti-AChE and BuChE activities and their inhibition kinetic were reported. In the present study, GMM and GMMG possess anti-AChE activity closely compared to the ethanol extracts of H. valentiae and G. edulis, with IC50 of 2.6 mg/mL and 3.5 mg/mL respectively (Suganthy et al., 2010). However, G. manilaensis did not show anti-BuChE activity compared to other red algae, postulating that the bioactive compounds that are responsible for anti-AChE activity in G. manilaensis are selective towards AChE but not BuChE. This finding revealed that G. manilaensis may have a lower tendency to cause toxicity compared to other red algae according to Jońca et al. (2015). BuChE is also known as plasma cholinesterase, which is most abundant in plasma, pancreas and liver (Jońca et al., 2015). Jońca et al. (2015) stated that “BuChE serves as the first line of defence to the toxic compounds that reach the bloodstream that might inhibit AChE, the sister enzyme that located in the nervous system” . Hence, G. manilaensis could be a weaker but safer anti-AChE agent compared to other algae.
Andriani et al. (2016) has reported hexanoic acid as the main contributor to the positive AChE inhibitory activity in their preliminary screening. In contrast, we have only discovered two fatty acids, 2-ethyl-dodecanoic acid and dioscoretine which do not have relevant activities to AChE and BuChE, besides dioscoretine for its hypoglycaemic effect (Loizzo et al., 2017). On the other hand, ML-236C, C16 Sphignosine and 2,6-Nonadien-1-ol in GMMG also do not contain neuroprotective and ChEs inhibitory activity. ML-236C, also known as (4R,6R)-6-[2-[(1S,2S,8aR)-2-methyl-1,2,6,7,8,8a-hexahydronaphthalen-1-yl]ethyl]-4-hydroxyoxan-2-one, is a carbobicyclic compound with anti-cholesteremic activity that was isolated from fungus Pythium ultimum (Endo et al., 1976). C16 Sphignosine is a phospholipid that possesses anti-bacteria (Reid et al., 2019) and anti-cancer activities (Bagheri et al., 2018). 2,6-Nonadien-1-ol is a volatile fatty alcohol that function as a flavour compound described in many papers (Api et al., 2015, 2019; Flath et al., 1983; Kemp et al., 1974; McGinty et al., 2010).
The five neuroprotective compounds discovered in GMMG were drawn to our attention. Graveolinine is the only compound in GMMG that has been reported to positively inhibit AChE (Luo et al., 2020). Besides, graveolinine and its derivative also demonstrated neuroprotective activity against the neurotoxicity of β-amyloid and scopolamine (Luo et al., 2020). Meanwhile, cyneirne A (Marcotullio et al., 2006; Obara et al., 2007) and militarinone A (Küenzi et al., 2008; Riese et al., 2004; Schmidt et al., 2002) possess neurite outgrowth activity against PC12 cells. Interestingly, their respective derivatives, cyathane diterpene (Cao et al., 2018), as well as 4-hydroxy-2-pyridone (Schröder et al., 2015) and (+)-N-deoxymilitarinone A (Cheng et al., 2006) also displayed neurite outgrowth activity as their precursors. Curcumenol (Hamdi et al., 2015) and Eplerenone (Dong et al., 2012; Wang et al., 2020) are also neuroprotective towards neuronal cell lines and mouse brain. Curcumenol also shown anti-neuroinflammatory activity against LPS-stimulated murine BV2 microglia (Hsiao et al., 2020; Lo et al., 2015; Pintatum et al., 2020; Tanaka et al., 2008), whereby eplerenone shown anti-inflammatory activity to a variety of illnesses and conditions such as viral myocarditis (Xiao et al., 2009), heart-related inflammations (Chen et al., 2018; Łabuzek et al., 2014; Suzuki et al., 2006), renal inflammation (Bayorh et al., 2012; Chen et al., 2013) and obesity-associated inflammasome (Vecchiola et al., 2020; Wada et al., 2017). These compounds made GMMG a very promising neuroprotective fraction to be used as nutraceutical agents for neuronal health.
Our findings disclosed that GMM and GMMG of G. manilaensis possessed inhibitory activity to AChE but not BuChE. GMM and GMMG showed mixed inhibition and uncompetitive inhibition to the AChE activity respectively. The anti-AChE activity of GMMG is likely to be contributed by graveolinine. Taking together, graveolinine from G. manilaensis can be a promising lead compound for the development of anti-Alzheimer medicine in future. However, it is crucial to further validate the cholinesterase inhibitory activities of G. manilaensis in animal cell culture in vitro and in vivo assays.